What is a Lithium Polymer Battery?
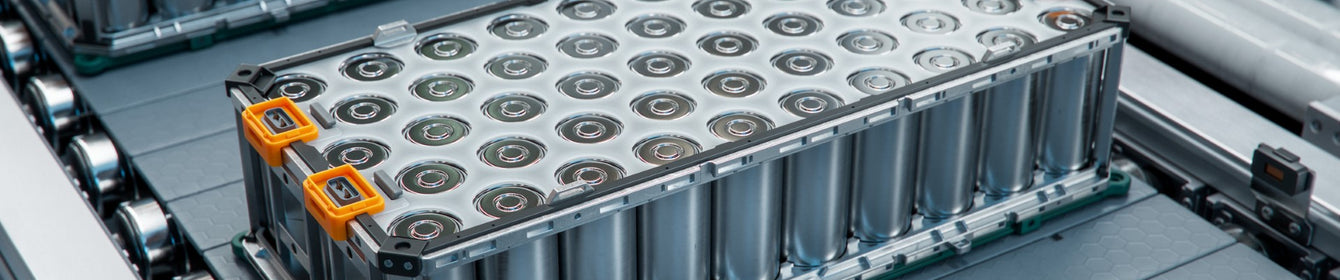
Lithium-polymer (LiPo) batteries are a type of lithium-ion battery technology that uses polymer electrolytes. This differs from standard lithium-ion batteries which use a solvent based electrolyte. Polymer electrolytes used are highly ionically conductive and form lithium-ion conduction channels. Polymer electrolytes were first used in dye sensitized solar cells but now are being investigated for use in batteries, fuel cells and more.
Polymer Electrolyte Properties
The key component of lithium-polymer batteries that differentiates them from other lithium-ion batteries is their polymer-based electrolyte. Typically a highly polar polymer that is capable of electron donation is used. Some key battery characteristics including energy density and the weight of batteries can be enhanced by using a polymer instead of traditional liquid electrolyte such as carbonate solvents.
Polymer electrolytes have undergone rapid progress in lithium-polymer battery development because of properties such as:
Low flammability
High ionic conductivity
Easy to process
Excellent thermal and electrochemical stability
Better electrode-electrolyte interfacial contact, resistance and compatibility than inorganic solid electrolytes
High Li-ion transference number
More tolerance to vibration, shock and mechanical deformation compared with liquid electrolytes
The ionic conductivity of polymer electrolytes is a crucial property for battery applications. It can be influenced by the surrounding environment of the electrolyte such as other components as well as the monomer units. Whilst ionically conductive additives such as salts are commonly used, non-ionic fillers can also improve a polymers ionic conductivity. High ionic conductivity along with high Li-ion transference number will lead to a reduced concentration polarization and an enhanced rate performance.
Crystallinity
The crystallinity of polymer electrolyte can severely reduce their ionic conductivity. In the non-crystalline amorphous phase, polymer chain motion creates a dynamic, disordered environment that plays a critical role in facilitating ion transport. In order to suppress the level of polymer crystallinity techniques including copolymerizing, cross-linking and grafting are used.
Types of Polymer Electrolyte
There are different types of polymer electrolytes used in lithium polymer batteries. They are differentiated by their physical state which in turn gives them different properties. The table below summarizes the different types of polymer electrolyte along with some key properties and advantages and disadvantages:
Characterisitics | Solid Polymer Electrolyte | Gel Polymer Electrolyte | Composite Polymer Electrolyte |
---|---|---|---|
Physical State | Solid | Gel (semi-solid) | Solid/Gel Mixture |
Ionic Conductivity / S cm-1 | 10-7 - 10-4 | 10-5 - 10-2 | 10-5 - 10-3 |
Energy Density / Wh kg-1 | 100 - 250 | 100 - 300 | 150 - 350 |
Power Density / W kg-1 | 100 - 400 | 100 - 500 | 150 - 600 |
Advantages |
|
|
|
Disadvantages |
|
|
|
Solid Polymer Electrolyte (SPE)
Solid polymer electrolyte is also known as solvent free polymer electrolyte. The solid polymer matrix usually contains inorganic salts which aid in the electrochemical performance of the electrolyte. The ratio of salt to polymer dictates the potential difference which influences the efficiency of charge transport between the electrodes via the electrolyte. Examples of polymers used are poly(ethylene oxide) (PEO), polyvinylidene difluoride (PVDF), polymethylmethacrylate (PMMA) and polyacrylonitrile (PAN). These polymers are used in combination with alkali metal salt complexes in order to be Li+ conductive material.
Coordination between Li+ and the Lewis basic sites of polymer chains results in the movement of Li+ being notably slower compared to anions. This is particularly apparent when comparing the ionic conductivity range for solid polymer electrolytes and gel polymer electrolyte. On the other hand, solid polymer electrolytes are much more stable and safe, with significantly reduced electrolyte leakage and flammability.
Gel Polymer Electrolyte (GPE)
Gel polymer electrolyte consists of a polymer matrix containing semi-solid polymer and liquid electrolyte also referred to as plasticizers, Li salts, and additives such as inorganic fillers. The polymers PEO, PAN, PVDF, poly(vinylidene fluoride-co-hexafluoropropylene) (PVDF-HFP), and poly(methyl methacrylate) (PMMA) have also been used in GPEs. Plasticizers are usually carbonates (propylene carbonate (PC), ethylene carbonate (EC), dimethyl carbonate (DMC), and diethyl carbonate (DEC)), ethers (tetraethylene glycol dimethyl ether (TEGDME), 1,2-dioxolane (DOL) and dimethoxymethane (DME)), and ionic liquids.
The gel matrix provides structural integrity while allowing the free flow of liquid electrolyte. This facilitates the high ionic conductivity observed, facilitating rapid charging and discharging. They combat issues faced by unsupported liquid electrolyte solutions by being less corrosive and less likely to leak.
Gel polymer electrolytes are prepared using physical or chemical methods. In the physical methods, GPEs are formed by dissolving polymer matrices in organic solvents and mixing them with inorganic fillers or other additives and then evaporating the organic solvents. Cross-linking of polymer chains forms through weak physical interaction between macromolecules. Chemical methods involve initiators, cross-linkers and monomers, dissolved in liquid electrolyte where the polymerization takes place. This "in situ synthesis" technique is simple, with high efficiency and low cost. However, there are issues of unreacted components behaving as impurities in the battery, causing decomposition and unwanted solid electrolyte interphase formation.
Composite Polymer Electrolyte (CPE)
Composite electrolytes are comprised of polymer matrices, inorganic fillers and lithium salts. The fillers can be non-ironically conductive (passive) fillers or ionically conductive (active) fillers. The conductive nature of CPEs are complex as multiple components can be ionically conductive. Composites can withstand high mechanical stress and strain, addressing issues of liquid electrolyte leakages.
Non-ionically conductive inorganic fillers including aluminium oxide (Al2O3) and silicon oxide (SiO2) nanoparticles have been used to increase the ionic conductivity of polymers. They enhance the electrochemical properties of the polymer, including the stability, by promoting the Lewis acid-base interactions between the surface groups of inorganic fillers and both the polymer chains and lithium salt anions. These filler particles are often described as ceramic. Ferroelectric ceramic fillers have been used to increase the dipole moment of polymer chains to enhance conductivity at the interfacial region. The surface charge of ferroelectric particles is likely to increase the amount of amorphous phase of the polymer, also increasing ionic conductivity.
Fast ionically conducting fillers are also used to increase the overall ionic conductivity and strength of the composite polymer electrolyte. A range of materials have been investigated including garnet-type (e.g., Li7La3Zr2O12 (LLZO)), NASICON-type (e.g., Li1.5Al0.5Ge1.5(PO4)3), and sulfides (e.g., Li10GeP2S12).
Single-Ion Conducting (SIC) Polymer Electrolyte
Single lithium-ion conducting polymer electrolyte involves polymers where anions are covalently bound so the only ion moving is lithium. Polymer electrolytes mentioned above are complexed with lithium salt, where both lithium cations and counter anions are mobile species. In general, anions move at least four times faster than lithium cations, as the motion of cations is highly coupled with Lewis basic sites in the polymeric host. As a result, lithium cation movement contributes only a small fraction (20%) of the overall ionic current.
By bonding anions to the polymer surface, they no longer collect at an electrode where they are not involved in a reaction in the way lithium cations are. This accumulation results in cell polarization and limited cycle life. Unwanted lithium dendrite formation is also avoided as lithium movement is even during charging/discharging cycles.
Lithium-Polymer Battery Advantages
There are many advantages of using polymer electrolyte in lithium-ion batteries, especially when comparing it to solvent electrolyte. These include:
- Improved Safety: Lithium-polymer batteries avoid the use of flammable solvent-based electrolytes, reducing the risk of leakage and fire.
- High Electrochemical Stability: Polymer electrolytes offer higher electrochemical oxidation resistance compared to many solvent-based alternatives, making them well-suited for high-voltage applications.
- Flexible Design: The inherent flexibility of polymers allows for easy lamination and stacking, eliminating the need for rigid packaging typically required by solvent-based systems.
- Enhanced Electrode-Electrolyte Adhesion: Polymer electrolytes provide better contact with electrodes, which improves overall battery performance
- Reduced Lithium-Plating Risk: Polymer electrolytes can suppress the formation of lithium dendrites, offering better protection for lithium-metal anodes and enhancing battery safety.
-
Material Versatility: A wide range of polymer and additive combinations can be used to tailor battery properties. For example:
- Ceramic-in-polymer electrolytes offer greater flexibility and lower cost, making them ideal for small-scale energy storage.
- Polymer-in-ceramic electrolytes provide higher mechanical strength and enhanced safety, better suited for large-scale batteries and electric vehicles.
Cathode Active Materials
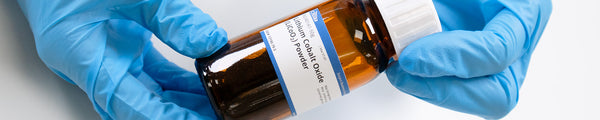
Learn More
Lithium plating is a mechanism of degradation in lithium-ion batteries (LIBs). It describes the accumulation of metallic lithium on the surface of the anode (usually graphite powder). Lithium ions gather at the anode surface and form metallic deposits.
Learn more...A solid electrolyte interphase (SEI) forms on the negative electrode in lithium-ion batteries (LIBs) due to the decomposition of electrolyte. The decomposition by-products build up on the surface of the anode and form an independent phase of material, different to the electrode and electrolyte.
Read more...
References
- Reducing crystallinity in solid polymer electrolytes for lithium-metal..., St-Onge, V. et al., communications materials (2021)
- Development of solid polymer electrolytes for solid-state lithium..., Li, J. et al., Materials today energy (2024)
- Polymer Electrolytes for Lithium-Based Batteries: Advances and Prospects, Zhou, D. et al., Chem (2019)
- Performance analysis of solid-state batteries in Electric vehicle..., Bobba, P. B. et al., E3S Web of Conferences (2024)
- Single-ion conducting polymer electrolytes as a key jigsaw..., Gao, J. et al., Chem. Sci. (2021)
Contributors
Written by
Application Scientist
Diagrams by
Graphic Designer