Fine-Tuning A-D-A Small-Molecule Acceptors for OPVs
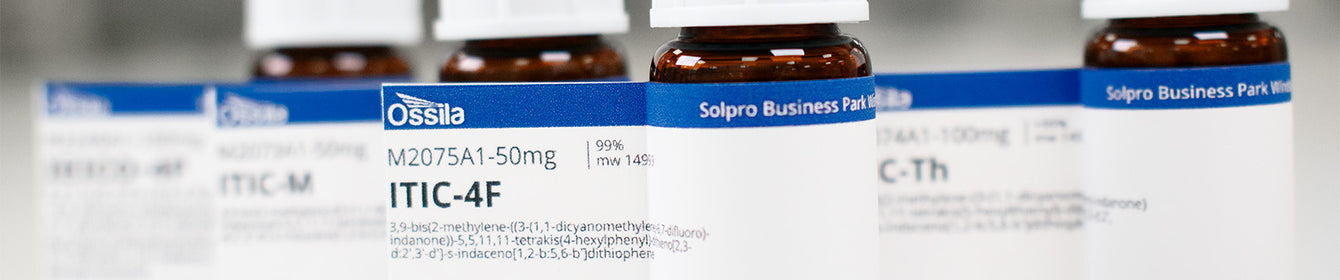
Small molecules such as non-fullerene acceptors1-4 are showing significant promise for application in organic photovoltaics (OPVs). Like OPV polymers, they have tunable properties such as band-gap width. Small molecules can be synthesised from basic chemical ‘building blocks’, and their properties can be tuned as desired by the addition of various chemical moieties, such as strong electron-donating or accepting groups.
Emma's comments: Small molecules can offer easier synthesis5 and processing over polymers, as they have less batch-to-batch variation (caused by variable polydispersity in polymers).6 However, it is also worth noting that in some cases, solution-processing small molecules gives less favourable film quality due to significant aggregation.7 This can be reduced through use of extended side-chains, as in ITIC.
What are A-D-A Small-Molecule Acceptors?
Many of the highest-performing small-molecule NFAs (including ITIC and IDIC) are based on an acceptor-donor-acceptor structure, or ‘A-D-A’, as shown in the figure below. Here, the donor (or ‘D’ part of the molecule) refers to an electron-donating unit; typically an indacenodithiophene (IDT) core; whilst the acceptor (or ‘A’ part of the molecule) refers to an electron-accepting unit;6 which is 2-(2,3-dihydro-3-oxo-1H-inden-1-ylidene)propanedinitrile (INCN) in both ITIC and the new NFA discussed here, NCBDT.
Emma's comments: Whilst IDT or a derivative is most often used as the core in small-molecule acceptors, there is also an interest in donors based on the A-D-A structure, often using a benzodithiophene core.7,8
Molecular Optimisation of Non-Fullerene Acceptors
When tuning the properties of a non-fullerene acceptor, the part of the molecule that is chemically modified will have a significant impact on the properties that are generated. Whilst the second article in this series discussed side-chain modification of the core, Kan and co-workers used a new fused-ring core structure derived from the basic ITIC form, therefore altering the donor part of the A-D-A structure. There has also been some success altering the acceptor end-capping group in other works.9
In earlier research,10 the authors designed an NFA with this new fused-ring core, known as ‘NFBDT’. To improve the performance of this via narrowing the band gap (and boosting JSC as a result), ‘NCBDT’ was generated by substituting the INCN acceptor unit with a highly electronegative fluorine atom. This is shown in the figure below, downshifting the HOMO and LUMO levels.
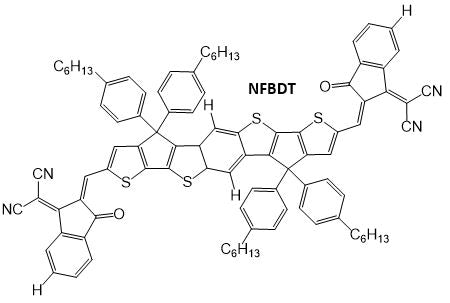
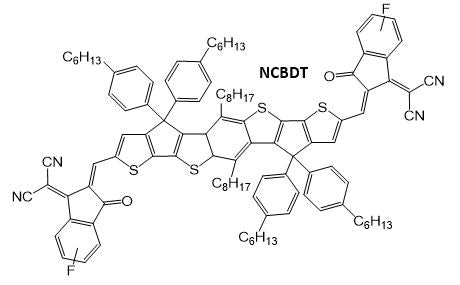
Similarly, an electron-donating alkyl group was introduced to the core to increase the donating ability of the donor unit. This also boosted the HOMO energy level and effectively narrowed the band gap, as illustrated in the figure below
Typically, the energetic offset between the HOMO levels of the donor and acceptor polymers within a bulk heterojunction is considered to be vital to drive charge separation. However, in the case of NCBDT, the reduction in energetic offsets compared to NFBDT appears to have little impact on the photo-physical processes of the acceptor. Both acceptors showed slow but efficient charge generation, and the independence from energetic offset means that both current and voltage losses can be optimised. Further discussion of the impact of energetic offset on photo-physical processes (in terms of the generation of interfacial charge-pair states) can be found within the paper itself.1
Emma’s comments: Typically, JSC and VOC cannot both be optimised, as improving one will come at the expense of the other. Generally narrowing the band gap, as done here, will increase JSC at the expense of decreasing VOC. This can be reduced through use of extended side-chains, as in ITIC.
In combination with a PBDB-T donor, the new NFA showed improved JSC and PCE in comparison to NFBDT, with slightly reduced VOC, as predicted through the chemical modification.

Final Thoughts
Using molecular optimisation to tune the band gap of small-molecule NFAs could be used to extend the absorption range of other acceptors, potentially boosting JSC. Recently, this has been used to great effect to give the record highest OPV PCE of 13.1%,11 using a non-fullerene acceptor, ITIC-2F. Kan and co-workers concluded that because of the independence of charge-separation efficiency from HOMO energetic offset, the balance between JSC and voltage loss could be optimised further through careful chemical modification, and that higher efficiencies may still be in reach. Further work optimising VOC12 and JSC13 can be found in recent literature. It is also worth noting that whilst small-molecule NFAs have become a significant area of research, small-molecule donors still lag behind their polymeric counterparts, and all small-molecule OPVs14 may offer processing advantages over the current material set used, in the future.
Materials Mentioned in This Paper
If you are interested in conducting a similar experiment, some of these materials are available from Ossila.
Non-fullerene acceptors
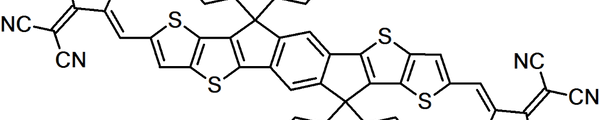
Learn More
References
- Fine-Tuning the Energy Levels of a Nonfullerene Small-Molecule Acceptor to Achieve a High Short-Circuit Current and a Power Conversion Efficiency over 12% in Organic Solar Cells, B. Kan et al., Adv. Mater., 1704904 (2017); DOI: 0.1002/adma.201704904.
- New developments in non-fullerene small molecule acceptors for polymer solar cells, N. Liang et al., Mater. Chem. Front. (1), 1291-1303 (2017); DOI:10.1039/C6QM00247A.
- Recent progress in non-fullerene small molecule acceptors in organic solar cells (OSCs), W. Chen et al., J. Mater. Chem. C (5), 1275-1302 (2017); DOI:10.1039/C6TC05066B.