Layer-by-Layer (LbL) Processing for Highly Efficient Organic Solar Cells
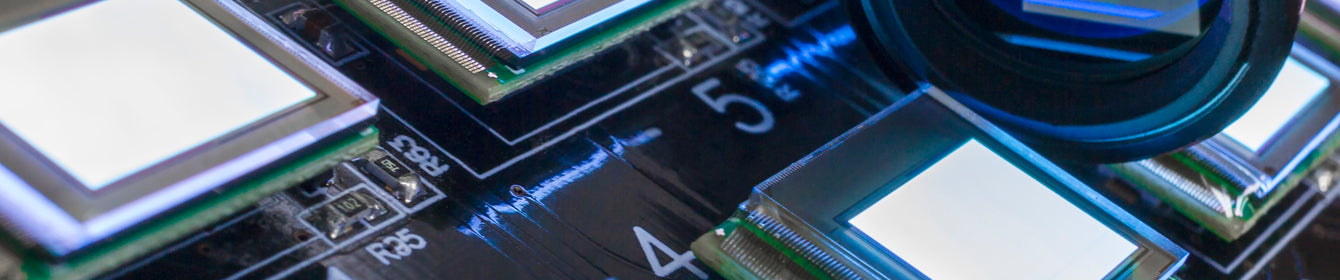
"Layer-by-layer" (LbL) processing, also known as "layer-by-layer" deposition, is a technique used for the fabrication of photovoltaic solar cells, in particular organic solar cells. This method involves the sequential addition of ultra-thin layers of materials to build up the device's structure. As a result, it allows for precise control over thickness, composition, and even chemical properties of each layer.
Layer-by-layer processed organic solar cells have emerged as a promising approach in photovoltaic technology. It is a unique construction process that optimizes morphology, power conversion efficiency, stability, and ultimately the device performance.
Layer-by-layer fabricated OSCs via sequentially processing the donor and acceptor layers have become a competitive option to achieve pseudo-bilayer configuration resembling the desired positive-intrinsic-negative (p-i-n) structure. It gives a favourable vertical phase separation, typically with an acceptor film cast on top of a polymer donor film. This construction ensures not only sufficient exciton separation on the interfaces but also provides independent transmission channels for the transport and collection of electrons and holes.
Key Points about LbL Processing in Solar Cells
The layer-by-layer (LbL) technique has been adopted for the processing for organic solar cells. There are a few key points about the technique that are worth noting:
Precision in Layer Thickness and Composition
- With LbL processing technique, each layer can be precisely deposited on top of each other with engineerable interface at nanometre or micrometre scales. This is essential for optimizing light absorption, charge separation, and transport within the cell.
- Precision deposition, morphology control and interface engineering enhance device efficiency and performance. It does this by minimizing defects that could cause changes in charge transport and carrier recombination. LbL processed active layers with precision in layer thickness and composition shows suitable vertical phase separation and higher crystalline domains that preserve the interfacial exciton harvesting and suppresses the charge recombination with reduced traps and defects.
Enhanced Interface Control
- LbL processing allows the fine-tuning of the interfaces between acceptor and donor layers. This is where most of the charge transfer and collection takes place.
- LbL sequential deposition approach can optimize the vertical phase separation which can enhance donor/acceptor interface area. This enable stronger photon absorption, facilitate charge transport, and suppress charge recombination in the active layers.
- The vertical active layer separation can be described as a three-phase morphology, where a substantial concentration of the donor can be found at the anode, acceptor at the cathode and a blend of donor and acceptor materials formed at the interface in the centre.
- Improved interfaces mean reduced energy losses due to recombination of charges recombine before generating current and thus increased efficiency and device stability. LbL film with ideal interface contacts is conductive to reduce the trap-assisted recombination, thereby improve the fill factor (FF) and power conversion efficiency (PCE).
Versatility and Customization
- LbL techniques are suitable for a wide range of materials, including organic and inorganic semiconductors, semiconducting polymers and small molecules, perovskites, quantum dots, carbon and metal oxide nanomaterials that can be processed either in solution by different solution deposition techniques or vacuum thermal deposition.
- Each layer's properties can be customized and optimized with either binary, ternary or tandem active layer structures to engineer unique morphological, optical and electrical properties.
Example Materials
Why Layer-by-Layer Processing?
In traditional bulk-heterojunction (BHJ) OSCs, donor and acceptor materials are mixed in a single step, which can lead to issues such as uncontrolled morphology, phase separation, and suboptimal charge transport. The layer-by-layer method, on the other hand, involves sequential deposition of donor and acceptor layers, allowing precise control over thickness, morphology, and the interfacial properties of each layer. This technique reduces the likelihood of large phase separation, enhances exciton dissociation, and promotes better charge transport.
LbL processing offers benefits like improved performance, enhanced control over the cell architecture, and increased stability. By stacking layers with precise control, it enables the creation of highly efficient solar cells with advanced functionalities, especially valuable in research and development of next-generation solar technologies.
Benefits of Layer-by-Layer Processing
There are some key benefits to using the layer-by-layer approach, especially when compared to bulk heterojunction processing. The sequential deposition technique provides the following important benefits:
Improved Morphology Control
With LbL sequential deposition, each layer can be optimized independently, reducing phase separation and improving material stability. For the construction of the BHJ solar cells, the miscibility between donor and acceptor, domain size and purity, molecular orientation, crystallinity and the vertical component distribution are the deciding factors for the ultimate performance efficiency of the device. Even though in the BHJ solar cells, sufficient interfaces are formed in the interpenetrating network structure for exciton separation, the thermodynamically formed BHJ morphology via casting the blend solution is inadequate for achieving suitable vertical component distribution and effective charge transfer and collection. LbL sequentially processed the donor and acceptor layers give a pseudo-bilayer configuration, which is similar to the desired p-i-n like structure of D/D:A/A, an interface of mixed donor and acceptor layer sandwiched between the donor and active layers. Such configuration with a favourable vertical phase separation ensures not only sufficient exciton separation interfaces but also provides independent transmission channels for the transport and collection of electrons and holes.
Enhanced Exciton Dissociation
By arranging donor and acceptor layers with optimized interfaces, layer-by-layer processing facilitates more efficient exciton separation. The diffusion distance of excitons is in the range of 5 – 20 nm in most of the organic semiconductor materials. To obtain sufficient exciton diffusion and dissociation, the donor and acceptor need to form phase separation domains with a size of ∼ 20 nm. The phase separation structure and miscibility between the active layer components have a pronounced effect on the exciton dissociation and charge transport process. Compared with the BHJ OSCs, sufficient donor/acceptor interface and independent charge transport channels can be simultaneously achieved via manipulating the component diffusion in the LbL structure.
Better Charge Transport and Collection
Control over layer thickness and morphology enhances charge mobility and reduces recombination losses, improving device efficiency. In principle, the energy disorders for both charge transfer and transport states have a great impact on charge transport and collection. In conjugated polymer semiconducting donors, the torsion and flexibility in the main backbone enlarge the energy disorder state. Furthermore, the introduction of acceptors into the donor phase further increases that disordered energy state. The reduced interface in pseudo-bilayer heterojunctions processed by LbL deposition are expected to reduce energy disorders of the charge transfer state formed at the donor/acceptor interface, the trap densities, and nonradiative recombination rates.
Better Stability
Devices processed by LbL deposition offers long-term photostability and thermal stability while comparing to the traditional bulk heterojunction devices where strong phase aggregation has been a common problem. LbL films form ordered morphology during the sequential coating process, maintaining the initial crystallinity and ordered packing of semiconducting donors.
Techniques Used in LbL Processing
The LbL processing techniques, operating procedures and choice of solvent has a striking effect on the thermodynamic morphology, optical and electrical characteristics of the active layers. For example, spin-coating has a radial effect on the cast films which have huge impact on the interface of different layers with the control of solution concentration, coating time and spin speed. Typically, LbL films can be achieved via two dominant approaches, including vacuum deposition and solution processing. Common techniques for LbL processing include:
Spin Coating
Spin coating is a universal and useful technique for applying thin and uniform layers. The challenge for spin coating solution lies in the finding of proper orthogonal solvents to avoid erosion of the lower layer from the sequential solution casting. “Orthogonal solvent” refers to a solvent that can well dissolve the upper layer for solution processing but remain insoluble or slightly soluble for the lower layer film. In most cases, dichlorobenzene (DCB) and dichloromethane (DCM), chlorobenzene (CB) and chloroform (CF) as orthogonal solvents to prepare quasi-bilayer solar cells based on fullerene and non-fullerene acceptors (NFAs), respectively.
Doctor-blade Coating
The doctor-blade coating technique, in combination of LbL solution processing, has attracted considerable attention due to its outstanding performance with simple structures and great potential for large-scale and large-area processing. LbL blade coating can achieve more thermodynamically favourable nanomorphology, with suitable donor/acceptor interfaces and larger separation of donor/acceptor domains. A polymer organic solar cell based on the star active layer combination of polymer donor PM6 and non-fullerene acceptor Y6, fabricated by doctor-blade LbL processing technique shows a PCE of 16.35%. This is close to the efficiencies of devices produced in the lab fabricated by spin-coating.
Physical Vapor Deposition (PVD) or Chemical Vapor Deposition (CVD)
Chemical vapor deposition is a great technique for the deposition of highly controlled thin-film layers, especially in the case of silicon-based or tandem solar cells. The LbL device prepared by the vacuum deposition method can avoid the solvent corrosion of the front layer, preventing the unnecessary blending of the donor and acceptor molecules. However, CVD is not particularly well working with semiconducting polymers and mainly suitable for the OSCs based on small-molecular materials. While P3HT can be thermally evaporated under high vacuum, significant molecular weight loss occurred during the deposition process due to the cleavage of side chains from the conjugated backbones.
Performance Characteristics of Layer-by-Layer Processed OSCs
The performance of OSCs is commonly evaluated based on parameters like open-circuit voltage (VOC), short-circuit current density (JSC), and fill factor (FF), resulting improved power conversion efficiency and enhanced device stability. Layer-by-layer processed OSCs have demonstrated efficiencies over 20%, especially when non-fullerene acceptors are used. This improved efficiency is the result from the enhanced exciton dissociation and charge transport due to the controlled morphology with vertical phase separation.
Additive-assisted layer-by-layer deposition produces a interpenetrating fibril network with a bulk inverted p-i-n feature and proper vertical segregation in the active layers. Induced by radial flow during spin-coating process, the interpenetrative fibril network also gives a wrinkle-like pattern in the active layers. Coupled with the reflective back electrode to increase diffuse reflection, the fibril morphology of the interpenetrative active layers enhances light capture capability. Furthermore, the gradient fibrillar morphology balances material interaction and crystallization at the interface. Exciton and carrier diffusion is promoted due to short fibrillar lengths (tens of nanometre scale) resulting in a reduction of recombination losses.
Unprecedented PCE of 20.8% was achieved for small-area devices based on a binary system of D18-Cl (donor) and BTP-4F-P2EH (acceptor) with the additive of 1-chloronaphthalene (CN). The device also maintained excellent stability after continuous heating for 400 h and light exposure for 500 h. The device was processed by LbL technique, using chlorobenzene (CB) for the spin coating of polymer semiconductor D18-Cl and then chloroform (CF) as the second solvent for the spin-coating of the non-fullerene acceptor BTP-4F-P2EH.
Solid additives tackles problems caused by the over-aggregation of NFAs such as Y6. As a result, the long-term stability device morphology is enhanced. For example, adding a solid additive of fatty acid (FA-C12) into polymer donor PM6 solution introduces controllable pre-phase separation. This allows for the enhanced diffusion of acceptor Y6 into the donor PM6 during the LbL processing. The fatty acid solid additive-assisted layer by layer (SAA-LbL) processing of active layers gives a controlled morphology with refined phase-separated domains and vertical phase-separation structure to better balance the charge transport and exciton dissociation. As a result, the binary single junction OSCs based on PM6/Y6 active layers demonstrate champion power conversion efficiency of 18.16% with SAA-LbL processing. With the same device processing technique, PCE of 19.02% is achieved for binary OSCs based on PM6/L8-BO active layers. All the SAA-LbL fabricated solar cell devices are processed with the same solvent of chloroform.
Summary
Layer-by-layer processed OSCs present a controlled approach to optimizing device morphology, improving charge transport, and enhancing efficiency and stability in organic solar cells. This method overcomes some limitations of traditional bulk heterojunction approaches, such as phase separation and recombination losses, by allowing tailored deposition of each layer. While challenges like solvent compatibility and scalability remain, continued research in materials science and process engineering could address these issues. Layer-by-layer OSCs hold promise for low-cost, flexible, and efficient solar energy solutions, paving the way for diverse applications and contributing to the growing demand for renewable energy technologies.
Non-fullerene acceptors
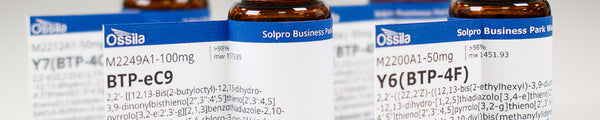
Learn More
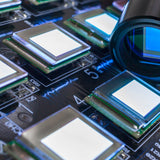
Organic photovoltaics (OPVs) have become widely recognized for their many promising qualities. This page introduces the topic of OPVs, how they work and their development.
Read more...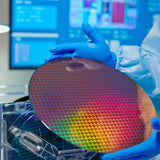
Organic semiconductors are materials, ranging from small molecules to polymers, that can transport charge. Unlike in conductors, where electrons move freely across the material, organic semiconductors rely on a structure primarily composed of carbon and hydrogen atoms.
Read more...References
- Sun et al. (2020); A Layer-by-Layer Architecture for Printable Organic Solar Cells Overcoming the Scaling Lag of Module Efficiency, Joule, 4 (2), 407-419; DOI: 10.1016/j.joule.2019.12.004.
- Yu et al. (2022); Layer-by-layered organic solar cells: Morphology optimizing strategies and processing techniques, Aggregate, 3, e107; DOI: 10.1002/agt2.107.
- Zhu et al. (2024); Achieving 20.8% organic solar cells via additive-assisted layer-by-layer fabrication with bulk p-i-n structure and improved optical management, Joule, 8, 1-16; DOI: 10.1016/j.joule.2024.08.001.