What is a Photodetector?
A photodetector is a device that can detect light, or more specifically photons. They are classed as optoelectronic devices like photovoltaic devices. This is because they produce an electronic signal which is proportional to the incident optical input. They rely on material components that can be photo-excited to produce charge carriers after photon absorption which then generate a current or voltage as a readable response. Photodetectors have four fundamental processes:
- Light harvesting
- Charge carrier generation (and separation)
- Charge carrier transport
- Extraction of charge carriers for output of signal current/voltage.
Traditional photodetectors based on inorganic III-V semiconductors exhibit excellent performance in terms of photosensitivity, responsivity, and detectivity. This is due to the high carrier mobility, good stability, and small exciton binding energy of such inorganic materials like silicon. Current research into photodetectors has expanded into using other semiconducting materials from 2D graphene and MoS2 to organic molecules and polymers.
On This Page
Photodetector Structure and Components
The structure of a photodetector is dependent on the type of photodetector and it's given application. The most important part of the photodetector is the same in any given device, the light-sensitive region. Below describes the key layer for any photodetector and other optional layers depending on the device.
Key layer
- Active layer - Photoactive semiconducting material capable of absorbing light across a range of wavelengths, from infrared to gamma rays, sometimes selectively. Photon absorption leads to the generation of charge carriers which can be harnessed to produce a measurable current. Material selection is dependent on the desired application.
Other layers
- Electrodes – capable of attracting the charge carriers
- Substrate - supports the device, can be conductive
- Charge Transporting Layers - help ensure efficient charge transport
- Dielectric Layer - used in some field-effect type phototransistors
- Passivation Layer - minimizes charge recombination and provides some protection to other layers
Types of Photodetector
There are many types of photodetector that are based on the absorption of a photon by an photoactive material and then the generation of a current. A brief summary of the different types of photodetector and their components are listed below:
Photodiodes
Photodiodes are semiconductor devices with a p-n junction or p-i-n structure (i = intrinsic material). Light is absorbed in a depletion region generating an exciton (electron-hole pair) which then separates into charge carriers. A particularly sensitive type of photodiode are avalanche photodiodes which have been used for photon counting.
Charge transport layers help to achieve high efficiencies by increasing the speed and efficiency at which charge carriers reach their respective electrode. Photodiodes are similar to solar cells but focus on photon absorption rather than current generation.
- Back electrode
- Charge transport layers
- Active layer
- Transparent electrode
Metal-semiconductor-metal (MSM)
Metal-semiconductor-metal (MSM) photodetectors contain two Schottky contacts rather than a p-n junction. The fork like metal-semiconductor junction exhibits rectifying behavior as it allows current to flow more easily in one direction than the other. It is heavily reliant on the work function of the metal and semiconductor used. MSM photodetectors have the potential to be faster than photodiodes.
- Metal electrode fingers
- Active layer
Phototransistors
Phototransistors are set up similarly to bipolar junction transistors (which use a p-n-p or n-p-n junction) or unipolar field-effect transistors. The signal produced by the photoactive layer can be amplified using the gate electrode, which allows for precise control and enhanced signal output. This amplification makes phototransistors highly sensitive, enabling them to detect even low-intensity light effectively. However, the amplification process can introduce delays, which reduces response times and makes them slower than photodiodes.
In devices with a unipolar active layer, a dielectric layer is included to improve the efficiency of charge separation and control. The layers work together to convert light signals into electrical currents efficiently, making phototransistors valuable in a range of applications, from optical sensors to signal processing in optoelectronic systems.
- Three electrodes:
- source
- drain
- gate
- Active layer - biopolar or unipolar
- Dielectric layer - if unipolar active layer
Photoresistors
Photoresistors (photoconductive detectors) have a simple structure with just the semiconducting photoactive layer and electrodes. They operate based on the photoconductive effect, where the conductivity of the material changes in response to incident light. When light strikes the active layer, it excites electrons, reducing the resistance and allowing more current to flow.
Photoresistors are cheap and can respond to long-wavelength IR but are slower than other photodetectors, not sensitive, and give a nonlinear response.
Phototubes
Phototubes are vacuum tubes or gas-filled tubes where the photoelectric or photoemissive effect is exploited. It is different to most other photodetectors as the cathode is typically the photoactive component. The photocathode emits electrons when struck by photons. The emitted electrons travel through the vacuum of the tube and are collected by the anode, creating a current. The work function of the photoactive materials is crucial to optimizing sensitivity of the phototube to a specific wavelength range.
- Typically photoactive cathode (photocathode)
- Thin anode
- Vacuum/Gas environment
Photomultipliers (PMTs)
Photomultipliers are a special kind of phototube, exploiting electron multiplication processes to increase responsivity. The electrons emitted by the photocathode are multiplied through a series of cascaded dynodes. Each dynode produces more electrons than the incoming electrons. They can also have a high speed and large active area.
- Photocathode
- Focusing Electrode
- Dynode
- Vaccum/Gas Environment
- Anode
CMOS Image Sensors (CIS)
CMOS image sensors are based on complementary metal-oxide-semiconductor (CMOS) technology. CIS devices integrate photodetectors and signal processing circuitry in a single pixel so it has its own charge-to-voltage conversion. CMOS sensors can read multiple pixels simultaneously, allowing for faster readout. They have low power consumption, high integration, and compatibility with standard CMOS fabrication processes.
Charge-Coupled Devices (CCDs)
Charge-coupled devices (CCDs) are image sensors composed of an array of pixels (tiny capacitors). Charge is generated in each pixel that is proportional to the intensity of light it is exposed to. The accumulation of charge in each pixel is sequentially transferred to an output amplifier. The final charge is converted to a voltage and then digitized to form an image. They are typically highly sensitive, with low noise and can capture a wide range of light intensities.
Key Physical Effects in Photodetectors
There are several physical effects that underpin photon detection mechanisms in photodetectors. The table below summarizes the key effects, their detection mechanisms, distinguishing features, and the devices that utilize them:
Effect | Mechanism | Key Feature | Application |
---|---|---|---|
Photoelectric |
Photon ejects electron from surface |
Direct ejection of electrons Active material needs a low work function |
Phototubes, photomultipliers (PMTs) |
Photovoltaic |
Photon generates exciton (electron-hole pair) which produces DC voltage/current |
Converts light to electricity without external power |
Photodiodes (also solar cells) |
Photoconductive |
Photon generates exciton which increases conductivity |
Changes in resistance |
Light Dependent Resistors, photodiodes (in photoconductive mode) |
Photoconductive Gain |
Amplification via generating multiple carriers per photon |
Enhanced sensitivity |
Avalanche photodiodes, PMTs |
Thermoelectric |
Converts light to heat, then measured electrically |
Temperature-based detection |
Infrared detectors, bolometers |
Internal Photoemission |
Internal photon-assisted electron transition |
Charge carriers moved across a junction |
Some phototransistors, photodiodes |
Charge Generation (CCD) |
Accumulates charge in pixels for readout |
Sequential charge collection and readout |
Digital cameras, scientific imaging |
Polarization |
Changes in light’s properties or the way the active material responds to light |
Focuses on light’s polarization properties rather than its intensity or energy. |
Polarimetric sensors |
Which Materials are Used in Photodetectors?
Photodetectors for the visible and near-infrared spectral region are mainly based on silicon (Si) and indium gallium arsenide (InGaAs) alloys. For UV detection materials such as zinc oxide (ZnO) are used. More recently photodetector research has expanded into using 2D materials like graphene and transition metal chalcogenides and tunable organic materials like polymers and non-fullerene acceptors. A whole host of materials have been used in photodetectors including:
- Mercury Cadmium Telluride (HgCdTe)
- Indium Gallium Arsenide (InGaAs)
- Silicon (Si)
- Amorphous Silicon (a-Si)
- Cadmium Sulfide (CdS)
- Germanium (Ge)
- Gallium Arsenide (GaAs)
- Organic Materials - Semiconducting Polymers, Fullerenes and Non-Fullerene Acceptors
- Perovskite Materials
- Zinc Oxide (ZnO)
- Graphene Materials
- Transition Metal Dichalcogenides (e.g. MoS2)
- Quantum Dots
- Black Phosphorus
Some photodetector devices with a combination of these materials are able to achieve high detectivity and responsivity within a broadband spectrum. Heterostructures aim to exploited the advantages of each type of material to address limitations. Heterojunctions are a well established technique in organic photovoltaic research and has been inspirational to research into organic photodetectors.
Properties and Characteristics of a Photodetector
The properties and characteristic of a photodetector include:
- Sensitivity to a certain spectral region (with large absorption at that region)
- Broadband - a detector's ability to effectively respond to and detect light across a wide range of wavelengths.
- Responsivity – electrical signal obtained per optical power depending on the optical wavelength
- A/W (amperes per watt) for current output.
- V/W (volts per watt) for voltage output.
- Detectivity - ability to detect weak signals in the presence of noise. It is essentially a measure of the signal-to-noise ratio (SNR) normalized for a given detector area and bandwidth. Measured in Jones.
- Response time - the time required for a photodetector to go from 10% to 90% of final output
- Dynamic range - ratio of the highest and lowest measurable light levels
- Quantum efficiency - the number of charge carriers generated per photon absorbed
- Detection bandwidth - the square root of the area of the detector separated by the noise equivalent power
- Noise-equivalent power - required light power needed to generate a signal that is equivalent to the noise of the device
- Dark current – current flow in the device in a light deficient environment
- Linearity - produce an output signal that is directly proportional to the intensity of incident light (not always required)
- Work function of the photoactive material - if the photodetector is reliant on the photoelectric effect then the work function of the semiconducting material is crucial.
- Photogain - the ratio of the detected charge carriers per single incident photons
The detector must also be suitable for some range of optical powers - the magnitude of the dynamic range (typically specified as the ratio of maximum and minimum detectable power).
Inorganic vs Organic Based Photodetectors
Inorganic photodetectors are the current commercially available products. Organic semiconductors are the relatively new material being explored within photodetector research. The table below compares some of the key advantages and disadvantages of each type of material for application in photodetectors:
Advantages | Disadvantages | |
---|---|---|
Inorganic |
|
|
Organic |
|
|
Photodetector vs Solar Cell
Both photodetectors and solar cells absorb light (photons) and generate electricity but they have different aims and priorities:
Photodetector
Primary Aim: To detect and measure light intensity or other light properties.
Priorities:
- Sensitivity: Photodetectors are optimized to detect even low levels of light, often requiring high quantum efficiency.
- Speed and Responsivity: Many photodetectors are designed for fast response times to capture rapid changes in light.
- Spectral Range: Photodetectors can be tailored to operate across specific parts of the electromagnetic spectrum (e.g., UV, visible, IR).
- Signal Accuracy: Emphasis is placed on reducing noise and ensuring precise detection of light signals.
Solar Cell
Primary Goal: To convert light into electrical power efficiently.
Priorities:
- Power Conversion Efficiency (PCE): Solar cells are designed to maximize the conversion of absorbed sunlight into usable electrical energy.
- Durability and Cost-Effectiveness: Materials and morphology are optimized for long-term exposure to sunlight, with a focus on reducing manufacturing costs for large-scale deployment.
- Broadband Absorption: Unlike photodetectors that may target specific wavelengths, solar cells are optimized to absorb a broad spectrum of sunlight, from UV to IR, to maximize energy capture.
- Energy Storage and Output: Emphasis is placed on generating a stable, continuous current that can be stored or fed into power grids.
Applications of a Photodetector
Photodetectors in optical sensing or imaging systems are used for medical, security, and industrial applications. See the table below for examples of how photodetectors are used in a range of fields:
Optical Communication Systems | LIDAR (Light Detection and Ranging) | Consumer Electronics | Medical Imaging and Diagnostics | Environmental and Energy Systems | Scientific Research | Military, Defense, Security and Surveillance |
---|---|---|---|---|---|---|
Fiber optic networks High-speed data transmission Telecommunication systems |
Autonomous vehicles Driver assistance systems (e.g., automatic braking) Rain and light sensors for windshield wipers and headlights Gesture recognition systems 3D mapping |
Remote controls (infrared detectors) Ambient light sensors in smartphones Optical mice and touch screens |
Blood glucose monitoring Fluorescence microscopy |
Pollution detection Ultraviolet (UV) and infrared (IR) radiation monitoring Weather and climate studies Solar power monitoring Optical sensors for power grid monitoring |
Astronomy and astrophysics (e.g., detecting faint starlight) Spectroscopy Particle detection in physics experiments |
LADAR – laser detection and ranging Missile guidance systems Laser rangefinders Infrared imaging for night operations Motion detectors Night vision systems Biometric scanners (e.g., facial recognition) |
Non-Fullerene Acceptors
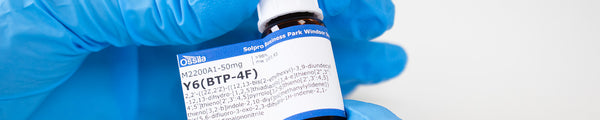
Learn More
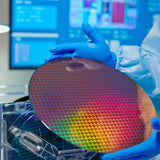
Organic semiconductors are materials, ranging from small molecules to polymers, that can transport charge.
Read more...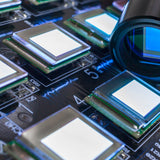
Organic solar cells have become widely recognized for their many promising qualities.
Read more...References
- Recent Progress in Organic Photodetectors and their Applications, Ren, H. et al., Advanced Science (2021)
- Reverse dark current in organic photodetectors and the..., Kublitski, J. et al., Nature Communications (2021)
- New insights into the ultimate performance of HgCdTe..., Kopytko, M. et al., Sensors and Actuators: A. Physical (2022)
- Progress in Infrared Photodetectors Since 2000, Downs, C. et al., Sensors (2013)
- Near-Infrared Sub-Bandgap All-Silicon Photodetectors: State of the Art..., Casalino, M. et al., Sensors (2010)
Contributors
Written by
Application Scientist
Diagrams by
Graphic Designer