An Introduction to Photoluminescence Quantum Yield (PLQY)
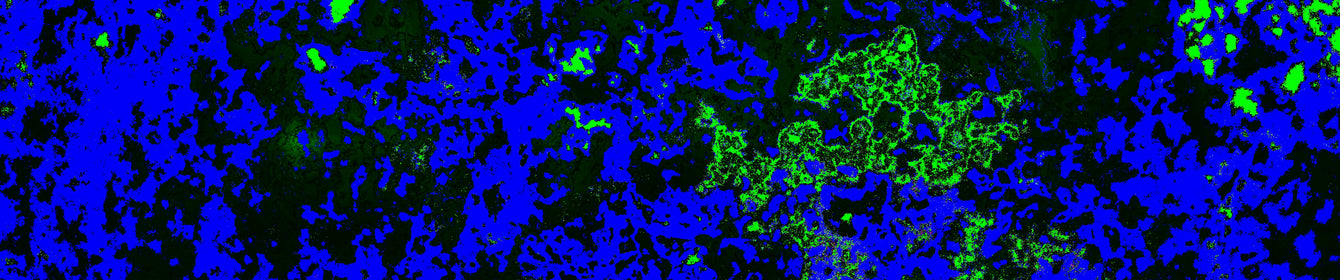
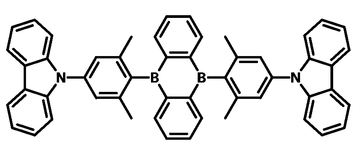
Photoluminescence quantum yield (PLQY) is a measure of the efficiency of photoluminescence in a system. It compares the number of photons emitted to the number of photons that have been absorbed. This essentially judges how well a photoluminescent material converts absorbed energy into emitted light. The emitted light can be fluorescence or phosphorescence depending on the material in question.
PLQY is a key indicator of a material's suitability for light-emitting applications. For instance, in organic light-emitting diodes (OLEDs), where efficient light generation is crucial, OLED materials must have high PLQYs. CzDBA has a reported PLQY of ~100% making it an ideal OLED material. The measurement provides insights into the extent of non-radiative processes in the sample that do not contribute to light emission.
How to Determine Photoluminescence Quantum Yield
There are two main ways to measure photoluminescence quantum yield:
Absolute PLQY Method
Photoluminescence quantum yield measurements use an integrating sphere fiber coupled with a USB spectrometer. The sample is prepared and placed in a cuvette positioned at the center of the sphere at a slight angle. This placement prevents direct reflection of light out of the entrance port. The sample is irradiated by a monochromatic light source, such as a laser, with photon energy higher than the sample’s emission energy. This ensures non-zero absorption at the incident wavelength.
The interior of the integrating sphere is coated with a diffuse white reflective layer, which distributes light isotropically through multiple reflections. Outgoing light, comprising both source photons and sample emission, is collected by an optical fiber for analysis.
To ensure reliable measurements, a control is required. For solutions, the control should be a cuvette with the solvent alone. For thin films, the control should be a blank substrate.
Comparative PLQY Method
The comparative method measures the light absorption rates and photoluminescence intensity of both a reference material and a target material. By comparing their spectra, the PLQY of the target material can be determined. While this technique was traditionally widely used, it has become less popular due to its drawbacks, such as limited access to suitable reference materials and the time-intensive nature of the process.
Photoluminescence Quantum Yield Calculations
Photoluminescence quantum yield (Φ) is calculated by dividing the number of photons emitted by the number of photons absorbed. The resulting number is presented as a percentage:
This data is gathered using the absolute or comparative PLQY measurement method. Using the absolute method as an example, the spectra from the sphere with and without the sample are overlayed. The PLQY of the sample is given by the emission area (light blue shaded area on the right) divided by the light absorbed area (dark blue shaded area on the left) (x100).
The PLQY of a material depends on its electronic and vibrational energy levels, which are dependent on molecular structure. When a photon is absorbed, the excited electron can either decay radiatively or non-radiatively:
- If it decays radiatively, a photon will be released and light is emitted.
- If it decays non-radiatively, however, a photon will not be released and light is not emitted.
The PLQY is hence related to the radiative (luminescence) rate, kr, and the sum (Σ) of all the non-radiative, knr, rates through:
Which Factors Influence Photoluminescence Quantum Yield of Materials?
Many factors influence the photoluminescence quantum yield (PLQY) of materials, which can be grouped into two main categories: external and material factors. External factors, such as excitation wavelength and sample environment, can affect the likelihood of non-radiative decay in a system. Similarly, material factors, including molecular aggregation, concentration, and purity, also play a significant role in determining PLQY.
External Factors
- Excitation Wavelength: The photon energy of the light source must be higher than the material's emission energy to ensure effective absorption.
- Solvent Polarity: The polarity of the solvent can significantly influence the photoluminescent properties of molecules by altering their electronic environment. Placing hydrophobic molecules in polar solvents can enhance aggregation effects and diminish PLQY.
- Sample Environment: Factors such as temperature, pressure, and the surrounding medium can affect non-radiative decay pathways. Interactions with other molecules, such as energy transfer or reabsorption of emitted light, can alter the PLQY.
- Sample Geometry and Placement: Proper alignment within the measurement setup ensures accurate light absorption and emission collection.
Material Factors
- Material Purity: impurities or defects in the material can introduce non-radiative decay processes, reducing PLQY.
-
Molecular Aggregation:
- Aggregation leads to broadened or shifted absorption and emission spectra, increases non-photoluminescent pathways, and reduces PLQY.
- Aggregation occurs when molecules form complexes in the ground state, altering their electronic properties.
- π-π stacking is a specific type of aggregation.
- Concentration of the Material: At high concentrations, self-quenching or aggregation-induced quenching can occur, reducing emission efficiency.
-
Solid-State Effects:
- In the solid state, close molecular proximity increases aggregation effects.
- If molecular distances are short enough, non-radiative energy transfer and intramolecular charge transfer can occur, further reducing PLQY.
- Surface Passivation: For nanomaterials like quantum dots, incomplete surface passivation can lead to surface trap states that facilitate non-radiative recombination.
OLED Materials
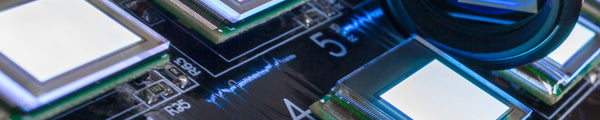
Learn More
Quantum efficiency refers to the fraction of input energy that is converted into useful output energy. It's different for different devices but is typically dependent on the photon to electron ratio (or other physical entity involved in an interaction).
Read more...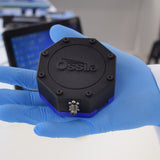
Photoluminescence refers to a form of luminescence that results from photoexcitation. Simply, photoluminescence occurs when a material emits light after absorbing a photon from an external light source and is measured using a spectrometer such as an optical spectrometer.
Read more...Contributors
Written by
Application Scientist
Diagrams by
Graphic Designer