The Properties of Carbon Nanotubes
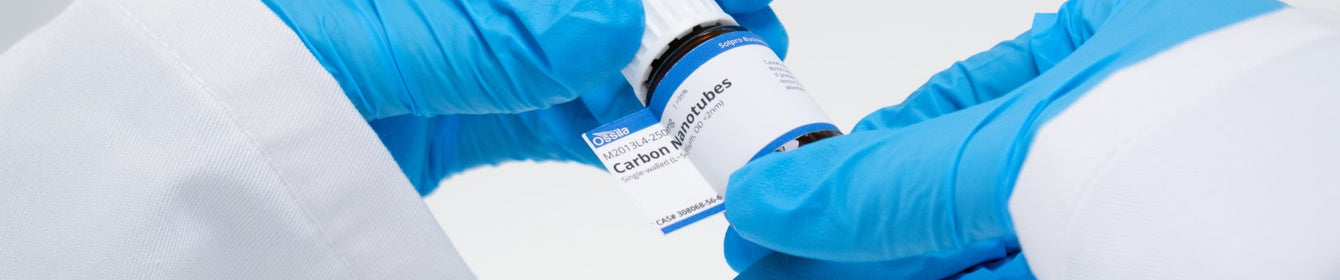
Carbon nanotubes (CNTs), like multi-walled carbon nanotubes (MWCNTs), have unique properties such as high conductivity and strength. They have similar properties to another carbon allotrope known as graphene. This is due to the similarity in structure of 2D sheet-like graphene and 1D carbon nanotubes which are essentially cylindrical tubes of rolled up graphene.
Structural Properties of Carbon Nanotubes
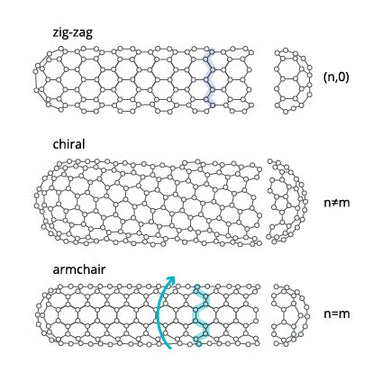
Carbon nanotubes get most of their physical properties from their structure, which is inherited from graphene. In both materials, carbon atoms are arranged in a regular sp²-bonded hexagonal pattern. The strength of these carbon-carbon bonds is crucial to many of their mechanical properties.
The key feature of carbon nanotubes is their one-dimensional cylindrical shape, formed by rolling a graphene sheet. Carbon nanotubes are nanomaterials with small diameters (nanoscale) and much longer lengths (micron scale). This gives them high aspect ratios with high specific surface area.
For single-walled carbon nanotubes (SWCNTs) the chirality of the nanotube is also important. It dictates the electronic and optical properties of the individual CNTs. SWCNTs can be:
- zig-zag
- armchair
- chiral
These names describe the orientation of the lattice, with different chiral index parameters (n,m). The armchair orientation induces metallic properties and zig-zag orientation induces semiconducting properties. This is further explained on our what are single-walled carbon nanotubes page.
With the structure of carbon nanotubes dictating properties, any defects from synthesis, handling or treatment can have a significant impact. Multi-walled carbon nanotubes are more resilient to defect formation than SWCNTs. The extra layers provide protection from compression.
Physical Properties of Carbon Nanotubes
The physical properties of carbon nanotubes are directly influenced by their structural properties. Carbon nanotubes have unique and desirable mechanical, electrical, thermal and optical properties. It is these properties that have seen CNTs being used in applications ranging from electronics to water purification.
Mechanical Properties - What makes carbon nanotubes so strong?
Property | Value |
---|---|
Young’s Modulus |
~ 1 – 1.28 TPa |
Tensile Strength |
~ 100 GPa |
Specific Strength |
48,000 kN m /kg |
Density |
1.3 – 1.4 g/cm3 |
The mechanical properties of carbon nanotubes mean they are often used to strengthen other materials. Their high specific strength and tensile strength are a result of the strong sp2 bonded carbon network. This allows them to provide significant support within composite materials. The one-dimensional structure of CNTs gives them fiber-like characteristics, enabling them to act as reinforcement within matrix materials such as polymers.
These fibrous properties of CNTs also extend to their behaviour under stress. When subjected to high force and compression, CNTs can bend, twist, kink and buckle. They undergo these deformations without getting damaged. Once the pressure is released, they return to their original structure.
Electrical Properties
Property | Value |
---|---|
Electrical Conductivity | 106 S m−1(SWCNT) 105 S m−1 (MWCNT) |
Theoretical Current Density |
4 x 109 A/cm2 |
Bandgap |
0 eV (metallic) ~ 0.5 eV (semiconducting) |
Resistivity |
10–4 ohm-cm |
The electrical properties of carbon nanotubes are dependent on the chirality of the hexagonal lattice. As mention in the structural properties section, SWCNT can have different orientations with different chiral index parameters. These lead to CTNs having either metallic or semiconducting properties depending on the bandgap. This means the different orientations are suitable for different applications.
The electrical conductivity of CNTs is extremely high, similar to graphene. There is a reduction in conductivity of a factor of ten going from SWCNT to MWCNT. This is due to inter-wall interactions, electron scattering and the misalignment of electronic properties of the different nanotube layers nestled within one another.
Thermal Properties
Property | Value |
---|---|
Thermal Conductivity |
0.1 - 6600 Wm−1 K−1 |
Phonon mean free path |
~ 100 nm |
Relaxation Time |
~ 10 – 11 s |
In carbon nanotubes, heat conduction is dominated by phonons, which are quantized vibrations of atoms in the lattice that act as heat carriers. The high thermal conductivity of carbon nanotubes is primarily due to the efficient phonon transport along the nanotube axis, as carbon-carbon bonds in CNTs are strong and form a rigid structure that efficiently transfers heat. Heat doesn't get conducted through CNTs evenly. Thermal conductivity is much quicker across the length of the nanotube than across the diameter. This is known as thermal anisotropy.
Phonons can travel exceptionally long distances (high phonon mean free path) without scattering, especially in the axial (length-wise) direction. This is because of the highly ordered, defect-free carbon lattice structure, which minimizes phonon scattering.
Optical Properties
Property | Value |
---|---|
Absorption |
UV, Vis and NIR |
Photoluminescence (excitation) |
NIR - 1100 – 1800 nm (700 – 1050 nm) (semiconducting SWCNT only) |
Raman SWCNT only |
RBM = ~ 100 – 200 cm-1 G band = ~ 1584 cm-1 G’ band = ~ 2600 - 2800 cm-1 D band = ~ 1300 - 1400 cm-1 |
The optical properties of carbon nanotubes are also heavily influenced by chirality. It determines whether the bandgap is present, which strongly influences their optical absorption, emission, and photoluminescence properties. As a result, optical properties can be tuned through control over the CNTs chirality.
Semiconducting SWCNT in particular have unique optical properties. The presence of a bandgap due to their lattice orientation means that they can emit NIR light upon photoexcitation. The wavelength of the emitted light is controlled by the orientation of the lattice so can be tuned to absorb and emit specific wavelengths of light.
No photoluminescence is observed for metallic CNTs as any holes generated by electron excitation are immediately filled by another free flowing electron. Inter-tube quenching interactions means that photoluminescence is also not observed with MWCNTs.
Raman spectroscopy can distinguish between single-walled and multi-walled carbon nanotubes through specific vibrational modes, such as the radial breathing mode (RBM), which is unique to single-walled CNTs. RBM frequency is inversely proportional to CNT diameter as it measures how to tubes oscillates (breathes). The G-band in Raman spectra reflects the CNT's electronic properties, helping determine whether a CNT is metallic or semiconducting. The D-band is associated with structural defects or disorder in the nanotube's carbon lattice. Its intensity relative to the G-band provides a measure of the defect density. Raman can reveal the diameter and chirality of CNTs, both of which are linked to their optical and electronic characteristics.
Multi-Walled Carbon Nanotubes (MWCNT)
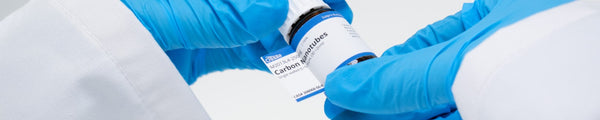
Learn More
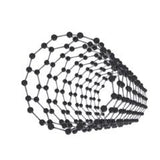
Carbon nanotubes (CNTs) have been deemed a wonder material due to their remarkable and highly unique physical and chemical properties. They have received much attention over the past decade as a promising material, particularly in the trending field of nanotechnology.
Learn more...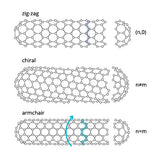
Single-walled carbon nanotubes (SWCNTs) are sheets of graphene that have been rolled up to form a long hollow tube, with wall thickness of a single atom. Their one-dimensional structure gives them extraordinary mechanical, electrical and thermal properties.
Learn more...References
- Carbon nanotubes: properties, synthesis, purification, and medical applications, Eatemadi, A., Nanoscale Res Lett. (2014)
- Understanding and Fine-Tuning the Mode of Interactions between..., Menon, A., Thesis (2021)
- Strength of carbon nanotubes depends on their chemical..., Takakura, A. et al., Nature Communications (2019)
- Carbon nanotubes for flexible batteries: recent progress and..., Zhu et al., National Science Review (2021)
- High-Field Electrical Transport in Single-Wall Carbon Nanotubes, Yao, Z., Phys. Rev. Lett. (1999)
Contributors
Written by
Application Scientist
Diagrams by
Graphic Designer