What is a Ternary Lithium Battery?
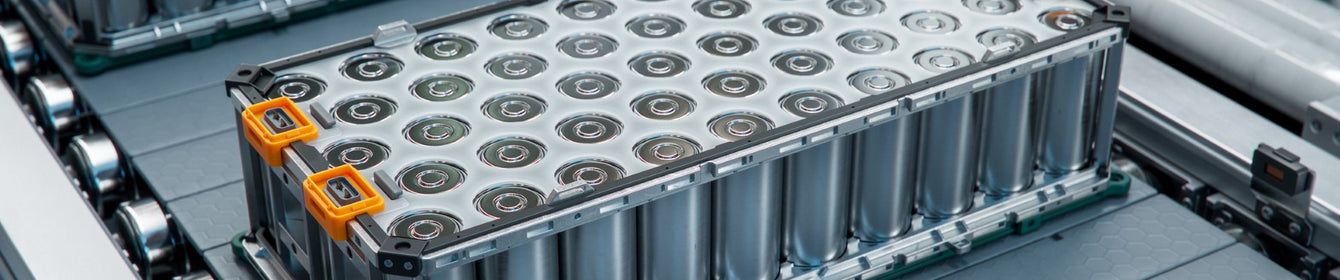
A ternary lithium battery is a type of lithium-ion battery (LIB) that has a cathode composed of three different metals. The metals are nickel (Ni), cobalt (Co), manganese (Mn) or aluminium (Al). The main ternary cathode materials are lithium nickel manganese cobalt oxide (NMC811 and NCM523) powder, and lithium nickel cobalt aluminum oxide (NCA) powder with the chemical formula:
NMC811 - LiNi0.8Mn0.1Co0.1O2
NCM523 - LiNi0.5Co0.2Mn0.3O2
NCA - LiNi0.8Co0.15Al0.05O2
Why These Metals?
Ternary lithium batteries get their name from the three metals that make up the cathode material along with lithium and oxygen. But why are these metals? Each one brings its own properties to the overal crystal structure. This means the properties of the crystal can be tuned through both the selection of the different metals and also the ratio the metals are in. The properties of each metal include:
Nickel - Usually the largest component by ratio. Enhances energy capacity by acting as the primary redox-active element. Its redox activity occurs at a higher potential compared to iron in lithium iron phosphate (LFP) or manganese in lithium manganese oxide (LMO), resulting in greater energy density. A higher nickel content increases the battery's capacity, making it a key component in high-performance lithium-ion cells.
Cobalt - Provides structural integrity to the cathode, stabilizing the layered structure of the material. This stability helps maintain battery performance over numerous charge-discharge cycles, preventing capacity degradation. It is more rare than nickel therefore is usually at a smaller ratio within ternary lithium batteries. It is also redox-active, contributing to a higher cathode voltage.
Manganese - Increases safety, stability and cyclability by reducing the risk of thermal runaway and reinforcing structural integrity. It provides outstanding rate capability, allowing for high current discharge while maintaining low temperatures.
Aluminum - Improves the thermal and structural stability of the cathode compared to manganese-based alternatives. Al–O bond exhibits strong polarity, weakening the Ni–O bond and increasing the working voltage. By strengthening the material, aluminum helps reduce unwanted reactions that could lead to degradation or safety risks.
Ternary Cathode Crystal Structure Comparison
Ternary lithium cathode materials are isostructural - they have the same structure. They are referred to as lithium transition-metal oxides and crystallize in the α-NaFeO2 structure belonging to R3m (D3d5) space group. This describes how the different metal oxides are arranged in space. All the metals exist as metal oxides where eight oxygen atoms are coordinated to the metal in an octahedral geometry. The d-orbitals of the metals overlap with oxygen's p-orbitals, forming strong, partly covalent metal–oxygen bonds. The different metal oxides share oxygen atoms via coordination to create layers of metal oxides. The different metals appear randomly in these metal oxide layers.
The lithium atoms are also coordinated to the oxygen atoms above and below these layers. Lithium's coordination to oxygen is weaker than that of the transition metals in ternary lithium cathode materials. This is due to lithium being small and monovalent, so it's interaction with oxygen is mostly ionic and relatively weak.
How do Ternary Lithium Batteries Work?
All lithium batteries, including ternary lithium batteries, work via electrochemical reactions at each electrode. Due to lithium's low reduction potential is is easily oxidised:
Li ↔ Li+ + e-
Charging
During battery charging, lithium atoms in the cathode are oxidized to lithium ions, which migrate through the electrolyte and intercalate into the anode material (typically graphite). Electrons released travel through the external circuit to the anode. The redox-active metal oxides within the ternary cathode also undergo oxidation, releasing electrons but remain coordinated to the oxygen atoms.
Discharge (battery use)
During discharge, the process reverses. Lithium ions deintercalate from the anode and travel back through the electrolyte to the cathode. Simultaneously, electrons flow from the anode to the cathode through the external circuit, providing electrical power to the device. At the cathode, the lithium ions reintercalate into the layered structure of the ternary metal oxide, and the redox-active metals are reduced, accepting the incoming electrons. This coupled ion and electron movement completes the electrochemical cycle and enables energy release in ternary lithium batteries.
The two key properties of transition metals that influence ternary lithium battery voltage and capacity are their redox potentials and valency:
Redox Potentials: Transition metals with higher redox potentials (can be reduced at a higher voltage compared to lithium) give you a higher cathode voltage. A higher voltage means more energy per lithium ion transferred between electrodes.
Metal Valency: Multivalent transition metals that can access multiple oxidation states have more electrons per metal. This means they have more capacity. For example, nickel can be oxidised from Ni2+ to Ni4+, giving two electrons per atom. This results in a higher specific capacity.
Not all the transition metals in the ternary cathode materials are redox-active. Whilst manganese is multivalent, it does not participate in redox reaction in NCM523 or NMC811. It doesn't therefore contribute to the battery's capacity but it does stabilize the structure.
During discharge, lithium is oxidized at the anode. The electrons released travel through the external circuit to the cathode. There, multivalent transition metals undergo reduction by accepting these electrons. Because they can exist in multiple oxidation states, they can accept more electrons. This increases the amount of charge the battery can store and deliver, resulting in a higher capacity.
Properties of Ternary Lithium Batteries
The properties of ternary lithium batteries are dictated by the properties of the transition metals and their ratio within the crystal.
High Specific Capacity – Due to high nickel concentrations more electrons can be transferred. The multivalent metal that can transfer multiple electrons. Allowing for longer runtimes and greater range in EVs.
Efficient Charge and Discharge Rates – Ensures consistent and reliable power output.
High Specific Energy – Specific energy combine both capacity and voltage, both of which ternary cathodes are optimized for.
Increased Voltage – The higher redox potentials of redox-active transition metals nickel and cobalt increase the cathode's voltage. As a result, the difference in electrochemical potential of the two electrodes is increased (if the anode remains the same). This difference defines how much energy per unit charge the battery can deliver.
Cathode Material | Average Discharge Potential | Notes |
---|---|---|
NMC523 | ~3.7 V | Good balance between energy and stability |
NMC811 | ~3.8 V | High energy, less stable, more sensitive to degradation |
NCA | ~3.7 V | High energy, similar to NMC811 but better thermal performance |
LFP (non-ternary) | ~3.2 V | Very stable, lower energy, high power output |
Challenges and Limitations of Ternary Cathode Materials
Ternary cathode materials offer high energy density, specific capacity and high cathode voltage. However, they also face several critical challenges in today's market, including structural instability, safety risks, raw material constraints, and increasing competition from alternative battery technologies. As lithium is extracted during charging, the cathode may undergo phase transitions from a stable layered structure (R3m) to a disordered spinel (Fd3m), and eventually to a NiO-like rock-salt structure (Fm3m) at the surface.
- Instability - One of the primary challenges in ternary cathode materials is structural degradation during cycling, particularly in nickel-rich compositions (NCM811 or NCA). Structural and thermal instability is due to the weaker Ni-O bonds, and thus a decrease in cycling ability. In addition, the chemical reactivity of the surface layer is increased due to the more oxidizing Ni3+/Ni4+ redox potential. Nickel atoms are at risk of leaving the transition metal oxide layer of the R3m structure and occupying the space left by lithium ions that have moved to the anode during charging.
- High Production Costs - The production of ternary cathode materials remains expensive, mainly due to the high cost of raw metals (nickel, cobalt, and in some cases, aluminum). Although raw material prices for lithium-ion batteries have generally declined since 2011, processing costs remain high, and the price of metals like cobalt has fluctuated sharply. The complex synthesis, purification, and quality control required for high-performance ternary materials also add to manufacturing costs, especially for high-nickel variants.
- Safety Concerns - Ternary cathodes, especially those with high nickel conten, are more susceptible to thermal runaway due to structural degradation and increased oxygen release at high states of charge. Nickel migration and structural collapse at the surface increase the likelihood of exothermic reactions, which can escalate under harsh conditions (overcharging, short circuiting). This makes thermal management and advanced battery management systems (BMS) essential when using ternary cathode chemistries.
- Competition from Emerging Technologies - Ternary cathode chemistries face increasing competition from:
- LFP (Lithium Iron Phosphate) batteries, which are cheaper, safer, and more abundant.
- Solid-state batteries, which aim to eliminate flammable liquid electrolytes.
- Post-lithium chemistries (e.g., sodium-ion, lithium-sulfur) that seek to avoid reliance on critical materials like cobalt or even lithium itself.
As manufacturers look for more sustainable, scalable, and safer alternatives, ternary cathode materials must continually improve in both performance and cost efficiency to remain competitive.
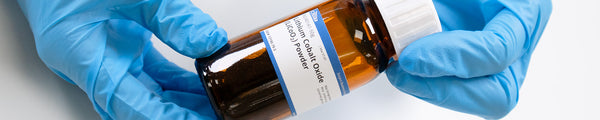
Cathode Active Materials
Learn More
The NCA battery gets its name from the cathode active material, lithium nickel cobalt aluminum oxide (LiNixCoyAlzO2, where x+y+z=1) which gets shortened to nickel cobalt aluminum (NCA). NCA is the cathode active material with a specific ratio of metals.
Learn more...Solid-state batteries (SSBs) differ from conventional lithium-ion batteries (LIBs) in terms of both their components and fundamental design features. Instead of a liquid electrolyte, they use a solid electrolyte to conduct lithium ions between electrodes.
Learn more...
References
- NCA, NCM811, and the Route to Ni-Richer Lithium-Ion..., Julien, C. M. et al., energies (2020)
- Lithium-ion battery fundamentals and exploration of cathode materials:..., Koech, A. K. et al., South African Journal of Chemical Engineering (2024)
- Safety Issues and Improvement Measures of Ni Rich..., Cui, B. et al., energies (2024)
- Study of the Failure Mechanisms of LiNi0.8Mn0.1Co0.1O2 Cathode..., Li, J. et al., Journal of The Electrochemical Society (2015)
Contributors
Written by
Application Scientist
Diagrams by
Graphic Designer