What is a Polaron?
A polaron refers to the local distortion of a molecular structure or lattice caused by a charge carrier moving through a semiconductor. It represents the combination of both the electron or hole and the displacement of atoms from their equilibrium position within the molecule or lattice. Polarons form easily in polarizable materials as excess charge carriers couple with ionic lattice vibrations, leading to localized lattice distortions.
Polarons are fermionic quasiparticles, which can be broken down as:
- Fermion – A subatomic particle that has a half-odd-integer spin (eg. spin 1/2) that obeys the Pauli exclusion principle (like electrons and holes)
- Quasiparticles – A group of particles, that undergo a collection disturbance or excitation within a material, can be treated as if they were a single particle (like excitons)
Theory Behind Polarons
Polarons form when atoms within a material or lattice are displaced from the position they would most commonly be found (equilibrium position). The displaced atoms are referred to as phonons. Electrons interact with the displaced atoms leading to interactions such as electron-phonon coupling. Other types of charge particle, such as holes or ions, can also interact with phonons following the same principles.
Atom displacement leaves a potential well in which the charge carrier sits. This is why its is often referred to as being "self-trapped". The polaron size and strength refers to the size and strength of the potential well.
Certain materials are more likely to host polarons due to their characteristics:
Material | Examples | Description of Polaron Formation | Name of Polaron |
---|---|---|---|
Polar Semiconductors | GaN, AlN, Perovskites | Charge carriers interact with polar phonons | Fröhlich polaron |
Ionic Crystals | NaCl, MgO | Charge carriers interact with ions | Holstein polaron |
Molecular Crystals | Organic Semiconductors | Charge carriers interact with molecular vibrations (molecular phonons) | Holstein polaron |
Polar semiconductors and molecular crystals are broad categories of materials. The interaction between charge carriers and phonons are heavily influenced by the material components and structure. The strength of polarons can be determined using models that take into account different influencing factors.
Polaron Models
There are many models that describe the behavior of polarons and their size/strength within a system. These models can be broadly divided into two main categories, depending on how they treat the interaction between the charge carrier and the surrounding lattice:
- Continuum models - Polarons are weakly or strongly coupled depending on whether the polaron binding energy is small or large compared to the phonon frequency. The discreteness of the crystal lattice is ignored so instead of treating the crystal as separate atoms it is considered a continuous medium.
- Lattice models – The strength of polarons depends on the relative size of the polaron radius compared to the lattice constant a (the length of one side of a crystal lattice unit cell). The crystal lattice is very much considered in this model of polarons.
When is a polaron small or large?
The size of a polaron is often related to the extent of the lattice distortion and therefore includes the number of displaced atoms or ions around the charge carrier.
- Fröhlich polaron (large): The radius of the polaron (potential well) is much larger that the lattice constant of the material. The mobility of a large polaron is often limited by scattering due to optical phonons. Large polarons experience wave-like propagation and weak electron-phonon coupling.
- Holstein polaron (small): The polaron (potential well) is approximately the volume of one unit cell or less. Electron-phonon coupling is strong and is not as free as a Fröhlich polaron. Small polarons have particle-like behavior with strong localization and discontinuous transport.
Polaronic Effects
Impeding Charge Transport
Polaronic effects are considered detrimental to the charge transport properties of semiconductors. The charge carriers are “self-trapped” as they move more slowly through the materials than free charged species due to the energy cost of maintaining molecular distortion as it moves. As a consequence, charge mobility—the ease with which charges move under an electric field—is reduced, which is particularly important in applications like solar cells, light-emitting devices, and field-effect transistors.
In organic materials, polarons typically hop between molecules due to the relatively weak overlap of electronic orbitals, further slowing charge transport compared to band-like transport in crystalline semiconductors.
Favorable polaron formation in Hybrid Perovskites
The charge-localized polaron state is energetically unstable without further stabilization. However, polaron formation in hybrid perovskites can be favourable. Electric dipole reorientation of the nearby cations minimizes the Gibbs free energy of the polaron. This reorientation lowers the overall energy of the system by stabilizing the local molecular distortion around the charge carrier, making it energetically favorable for the polaron to persist. In addition, the flexible lattice structure protects the charges from other ultrafast thermal relaxations. This property contributes to the high charge-carrier lifetimes and efficient charge transport observed in perovskites, making them excellent materials for solar cells and optoelectronic devices.
Balancing Polaronic Effects in Devices
While excessive polaron formation can lower charge mobility and reduce performance, moderate polaronic effects can enhance charge stability, prevent recombination, and contribute to high efficiency in soft semiconductors like perovskites. Device engineers often aim to strike a balance between minimizing detrimental polaronic trapping and leveraging beneficial polaron stabilization.
Triplet-Polaron Annihilation (TPA)
In organic light-emitting diodes (OLEDs) one of the degredation mechanisms is known as triplet-polaron annihilation (TPA). Triplet state excitons are harvested in OLEDs in order to achieve 100% internal quantum efficiency. However, the energy of a triplet exciton can be absorbed by a polaron. This demotes the triplet exciton to the ground state without radiative emission and promotes the polaron to a higher excited state. The higher energy polaron has the ability to break specific bonds in the molecules, causing fragmentation. This exaserbates the reduced charge mobility as the fragments also form charge traps.
Triplet-polaron annihilation can be reduced by increasing OLED thickness, introducing bulky substituents to separate molecules, and optimizing the layer design to spatially confine polarons and triplet excitons.
OLED Materials
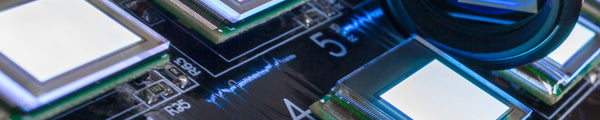
Learn More
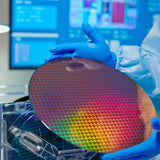
Organic semiconductors are materials, ranging from small molecules to polymers, that can transport charge. Unlike in conductors, where electrons move freely across the material, organic semiconductors rely on a structure primarily composed of carbon and hydrogen atoms.
Read more...The rapid improvement of perovskite solar cells has made them the rising star of the photovoltaics world and of huge interest to the academic community.
Read more...
References
- Polarons in materials, Franchini, C. et al., Nat Rev Mater (2021)
- Recent Progresses of Polarons: Fundamentals and Roles in..., Ren, Z. et al., Advanced Science (2023)
- Large and Small Polarons in Highly Efficient and..., Nandi, P. et al., Sol. RRL (2024)
- Excited-state vibrational dynamics toward the polaron in methylammonium..., Park, M. et al., Nature Communications (2018)
Contributors
Written by
Application Scientist
Diagrams by
Graphic Designer