What is a Chromophore?
A chromophore is a molecule or section of a larger compound that absorbs and reflects specific electromagnetic radiation. Any visible light that is reflected by the molecule is observed as colour. Radiation that is absorbed can activate ground state electrons in the chromophore into its excited state (if the energy is sufficient). This can induce conformational changes via electronic transitions.
Chromophore Structure
The structure of a chromophore heavily influences its resulting color. Functional groups that are able to absorb electromagnetic radiation above 200 nm are the most influential. Chromophores can be split into two groups depending on their orbital chemistry:
- Chromophores that only contain π electrons (in double bonds) undergo π-π* orbital transitions
- Chromophores that contain π electrons and nonbonding electrons (n). Nonbonding electrons are typically found as lone pairs of electrons on heteroatoms (N, S, O etc). These chromophores can undergo both n-π* and π-π* transitions.
When a chromophore is exposed to light of a specific energy an electron can be promoted from a bonding or non-bonding orbital into a higher energy, empty, anti-bonding orbital. This promotion is shown as arrows in the diagram below where the important transitions for chromophores are highlighted.
For chromophores, we are only interested in transitions that can occur as a result of absorbing light of wavelengths 200-800 nm. Therefore, only π-π*, n-π* and occasionally n-σ* transitions are of interest. Electron promotion for these transitions is possible from the absorption of light 200-800 nm. Each transition can occur via the absorption of light of different wavelengths depending on the surroundings of the orbitals involved in the transition. The wavelengths are indicated in the diagram below.
Chromophore Functional Groups
Most chromophore chemical groups are attached to conjugated molecules. These chromophore functional groups provide the orbitals that undergo key electronic transitions. Examples of related chemical groups include:
Group Name | Structure | Transition | Characteristics |
---|---|---|---|
Alkene | C=C | π-π* | π-conjugated |
Azo | N=N | n-π* | Dependent on surrounding moieties, π-conjugated |
Carbonyl | C=O | n-π*, n-σ* | Strong Electron Withdrawing, π-conjugated |
Carboxyl | COOH | n-π* | Acidic, pH dependent, π-conjugated |
Amide | CONH2 | n-π* | Electron-rich, π-conjugated |
Nitro | NO2 | n-π* | Strong Electron Withdrawing, π-conjugated |
Nitroso | N=O | n-π* | π-conjugated, Electron Withdrawing |
Nitrate | ONO2 | n-π* | π-conjugated, Electron Withdrawing |
The role of conjugation in chromophores
Many of the functional groups listed above have π-conjugation characteristics. This property plays a huge role in light absorption. For the conjugated π-system, electrons are delocalised and resonate throughout all the π bonding orbitals. By elongating the conjugated system, absorption shifts to longer wavelengths:
The shift in the absorbance spectra to longer wavelengths because the gap between the π-bonding and π*-anti-bonding orbitals decreases. These orbitals are also referred to as the highest occupied molecular orbital (HOMO) and lowest unoccupied molecular orbital (LUMO). This gap determines how much energy is required to promote an electron. The small the gap the less energy required to promote the electron. Therefore, a longer (lower energy) wavelength is absorbed.
The role of metals in chromophores
Metal ions are often found in inorganic and organometallic chromophores, coordinated to organic ligands. Metal centres in chromophores play a multifaceted role, including tuning light absorption, stabilising electronic configurations, enabling charge transfer, and promoting efficient emission processes. They allow further access to the tuning of light absorption through:
- Facilitating electronic transitions: Metal centres provide access to d-orbitals that participate in electronic transitions. These transitions typically involve movement of electrons (charge transfer) between metal d-orbitals and surrounding ligand orbitals (MLCT or LMCT), leading to light absorption in the visible or UV region.
- Modulate energy gap: The type of metal and its oxidation state can modulate the energy gap between electronic states, thereby shifting the absorption wavelength of the chromophore.
- Enhancing Stability and Delocalisation: Metal centres stabilise the electronic structure of the chromophore by coordinating with ligands, enhancing electron delocalisation across the system.
Auxochrome
An auxochrome is a chemical group attached to the chromophore which modifies the ability of the chromophore to absorb light. It does this via altering the wavelength or intensity of the absorption. By itself it does not absorb radiation in the near UV region. They are saturated and unsaturated groups that consist of one or more non-bonded electron pairs. An auxochrome influences the intensity of absorption of the chromophore as a whole molecule.
Auxochrome functional groups
Group Name | Structure | Transitions | Characteristics |
---|---|---|---|
Hydroxyl | OH | n-σ* | Polar |
Amine | NH2, NHR, NR2 | n-σ* | Polar, Basic |
Thiol | SH | n-σ* | Polar |
Tuning Absorption Maxima
There are two key ways in which to tune the absorption maxima of a chromophore:
- Change the conjugation length
- Change the strength of the electron donor/acceptor groups
- Introduce a metal ion
Increasing conjugation length is one way to increase the wavelength of the absorption maxima of a chromophore. Introducing difference functional groups with different electron donor and acceptor properties is another. These groups are also more sensitive to the sounding environment of the chromophore and can be used to tune responsiveness to certain conditions such as pH.
Metal ions bring access to d-orbitals and therefore different transitions. They allow the possibility of metal-to-ligand and ligan-to-metal charge transfer transitions which lead to light absorption in the visible or UV region. Different metals and their oxidation state will have different effects.
What color is observed?
The observed colour of a chromophore depends on the wavelengths of light it absorbs and the ones it reflects or transmits. When a chromophore absorbs light, it typically absorbs specific wavelengths corresponding to its electronic transitions. However, the colour we perceive is not the colour of the absorbed light but rather the combination of the remaining, unabsorbed wavelengths that are reflected or transmitted to our eyes.
For example, if a chromophore absorbs light in the blue region of the spectrum (around 450 nm), the complementary colour—orange or red—may be observed. This relationship between absorbed and observed colour is rooted in the concept of complementary colours on the colour wheel. The specific observed colour depends on which portion of the visible spectrum is absorbed most strongly and how the remaining wavelengths combine.
In practical applications, factors like the molecular structure, conjugation length, and surrounding environment of the chromophore can influence the exact absorption spectrum and, consequently, the colour observed.
Chromophore Examples
Naturally occuring chromophores
There are many naturally occurring chromophores in the world, responsible for the wide spectrum of colours seen in plants and animals. Examples of naturally occurring chromophores include chlorophyll, heme, and indigo:
Molecule | Structure | Absorbeh Wavelength | Observeh Color | Use |
---|---|---|---|---|
Chlorophyll |
Porphyrin ring system with a magnesium ion at the centre Chromophore groups |
Red (~660 nm) Blue (~430 nm) |
Green | Photosynthesis |
Heme |
Porphyrin ring with iron ion at the centre Chromophore groups |
Green and blue (~540-580 nm) | Red | Allows red blood cells to carry oxygen |
Indigo |
Conjugated π system Chromophore groups |
Yellow/orange (~620 nm) | Blue | Dye for denim fabrics |
Indigo, a natural dye found in the leaves of plants, is now used to dye clothes. The indigo molecule has a simple but highly conjugated structure with the common chromophore group carbonyl that can hydrogen bond with the secondary amine group to hold the molecule in a planar conformation. This conformation enhances the degree of conjugation of the molecule and electrons can easily flow through the π system.
Synthetic chromophores
Synthetic chromophores have been developed by researchers for various applications including, biological sensing and imaging, sunscreen, organic-light emitting diodes, solar cells, crime scene analysis and many more. By building on the knowledge collected by researching naturally occuring chromophores, materials can be designed using molecular engineering. Through the tuning of conjugation and different functional groups, absorption properties are dictated along with other molecular requirements for a given applications.
An example is the chromophore AQ(PhDPA)2 which has a large conjugated structure with carbonyl chromophore groups and tertiary amine auxochrome groups. This gives rise to an electron deficient anthraquinone centre and two electron-rich triphenylamine units. The push-pull effect of these chemical groups significantly contribute to the way the molecular interacts with light. In this case the molecule also emits light which is why it is used in organic light-emitting diodes.
Examples of Chromophore Applications
Chromophores are used in a huge range of applications across a spectrum of research fields. The way molecules interact with UV and visible light is of great interest. Understanding how chromophores function can provide solutions to some of the world’s most pressing challenges. Key areas of research include:
Organic Light-Emitting Diodes
Although the traditional definition of a chromophore focuses on light absorption, its meaning in OLEDs is broader. Traditionally, a chromophore absorbs light within the visible spectrum and produces colour through selective reflection of wavelengths. In OLEDs, the term now also describes the molecule’s role in both energy absorption and light emission. This shift reflects how light absorption and emission are linked in electroluminescent OLED materials.
The same conjugated π-electron system in the molecule governs both absorption and emission processes. This system determines the energy gap between the excited and ground states, which influences the colour of light emitted. Since the chromophore controls both processes, researchers use it as a general term.
Artificial Photosynthesis
Chlorophyll is an example of a naturally occurring chromophore. Molecules absorb blue and red light leaving green light unabsorbed and reflected so that is the color we observe of leaves. It is the structure of chlorophyll that has inspired the design of molecules for artificial photosynthesis to harness the suns energy. Chlorophyll molecules are made up of a conjugated molecular ring (porphyrin) that contains the heteroatom nitrogen that is functionalised with other chemical groups including carbonyl (ketone and ester) groups. All these components contribute to an absorption spectrum with two peaks, one 400-500 nm (blue) and another 600-700 nm (orange/red).
Chromophore microbiology
Chromophores are used in microbiology in various staining techniques to differentiate between types of microorganisms. As the dye is exposed to different environmental conditions the observed color can change. This allows the dye to be an indicator of specific environmental conditions. Chromophores used in staining microscopic samples are charged either positively or negatively depending on the chemical structure. The charged chromophore is attracted to the opposite charged species. Therefore, if a chromophore is positively charge, it is classed as a basic dye which tend to stick to negatively charge cell walls.
Examples of staining dyes used in microbiology:
- Positive Stain / Basic Dyes - crystal violet, malachite green, methylene blue, and safranin
- Negative Stain / Acidic Dye - acid fuchsin, eosin, and rose bengal
OLED Materials
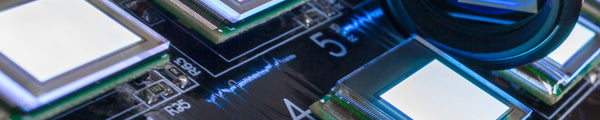
Learn More
Contributors
Written by
Application Scientist
Diagrams by
Graphic Designer