Phosphorescent Organic Light-Emitting Diode
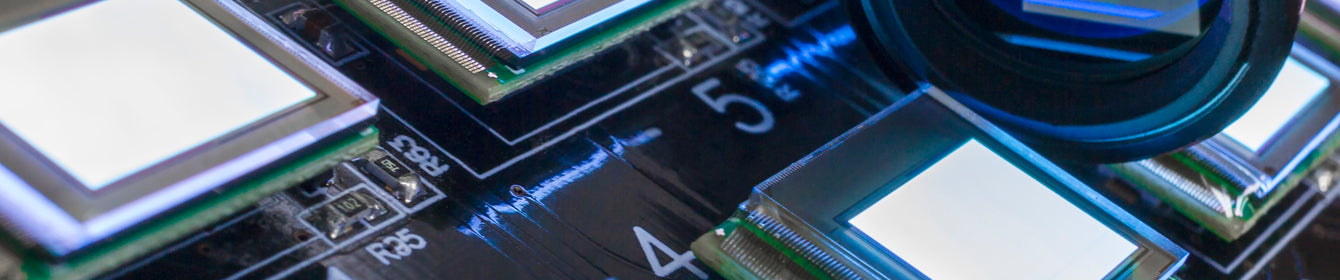
Phosphorescent organic light-emitting diodes (PhOLEDs), a subset of OLED electronic devices, feature photoactive layers that emit light through phosphorescence. They have recently gained significant popularity due to their superior energy efficiency compared to fluorescent based OLEDs.
In phosphorescent light-emitting diodes, charge carriers are injected from the electrodes into the organic layers, where they recombine in the emissive layer to radiatively emit phosphorescence. This emission can be tuned to specific wavelengths and colors through the selection of phosphorescent OLED materials. By altering the chemical structure of these components, it is possible to control the emitted light's color, ranging from deep blue to red. This tunability is essential for applications such as high-resolution displays and lighting systems.
Phosphorescent OLED materials represent the second-generation of OLED design. The mechanism by which excitons are harvested as light drives high device efficiency and a reduction in power consumption. This technology is driving innovative OLED research, opening new possibilities for broader color ranges and extended device lifetimes.
Phosphorescent Organic Light-Emitting Diode Structure
The structure of phosphorescent organic light-emitting diodes is the same as other OLEDs. The layers within the device remain the same, responsible for charge transport and/or light emission. The multi-layer architecture include electron and hole injection and blocking layers to increase device efficiency.
Whilst the structure of PhOLEDs is the same as any other OLED, the materials in the emissive layer are different. The mechanism of light emission requires materials that are capable of phosphorescence. The host and dopant materials within the emissive layer work together to produce highly energy efficient light emission.
Dopant Materials
Phosphorescent emitters generate light directly through phosphorescence. When excited, dopants release energy as light, determining the emission color of the device.
Host Materials
Host materials support dopants by facilitating energy transfer and managing charge transport. They absorb electrical energy and transfer it to the dopant, ensuring efficient light emission. To perform this function effectively, host materials must be capable of transporting both electrons and holes, making them ambipolar. A well-designed host minimizes energy loss, prevents quenching of the dopant's excited states, and enhances overall device performance.
Mechanism of Light Emission: Phosphorescence
For phosphorescent organic light-emitting diodes (PhOLEDs) the mechanism of light emission is phosphorescence. When the charged species (electrons and holes) are injected from the electrodes and reach the emissive layer they recombine and radiate emission in the form of light.
The key difference from fluorescence is that electrons in the excited state are able to undergo intersystem crossing to access the triplet state, where they can radiatively recombine through phosphorescence.
The injected electrons cause the organic molecules in the emissive layer to become excited (S1 or S2 etc.). The molecules are then designed to emit light of a particular wavelength when those electrons radiatively relax back to the ground state to recombine with the hole.
For phosphorescent molecules, electrons can reach the triplet state via intersystem crossing, which is a non-radiative process. This transition can occur due to spin-orbit coupling, as an electron has to flip its spin state (which is not spin-allowed). Once the electron is in the triplet state, it will eventually radiatively relax into the ground state (S0) or one of the vibrational states above.
Phosphorescent Materials for PhOLEDs
The materials used in phosphorescent organic light-emitting diodes (PhOLEDs) are meticulously designed to optimize performance and maximize light emission. Host and dopant materials collaborate to achieve efficient light generation and energy transfer in the emissive layer. The host materials requirements include:
The compatibility between the host and dopant materials is crucial, as their energy levels must align to maximize energy transfer and emission efficiency. The key parameters for phosphorescent emitters are:
To access phosphorescent materials, molecular engineers have focused on promoting the intersystem crossing (ISC) process. This can be done by incorporating different chemistries such as heavy atoms, heteroatoms and aromatic carbonyls to enhance spin-orbit coupling. Organic molecules can also be designed so that the energy gap between their singlet and triplet states is minimal, enhancing ISC rates. As well as this, the unwanted non-radiative decay of triplet excitons has been reduced by rigidifying materials and introducing host-guest assembly.
There are two main types of PhOLED emissive layer materials:
- Metal Coordination Complexes:
Metal complexes are commonly used as dopant phosphorescent emitters in PhOLEDs. These complexes consist of a core metal, such as Ir, Pt, Pd, Eu, or Tb, coordinated with π-conjugated ligands, which enable intense phosphorescence. Upon excitation, electrons can be found in metal-centred (MC), ligand-centred (LC) and charge transfer (CT) states. Electrons can access the low-lying triplet 3LC and 3CT states (MLCT, LMCT, LLCT) via internal conversion and intersystem crossing and radiatively recombine. MC states are responsible for non-radiative decay. Strong spin-orbit coupling between high-lying singlet states and the emitting triplet state mean these dopant materials are theoretically able to achieve electroluminescent quantum efficiencies up to 100%.
The spin-orbit coupling routes depend on the coordination geometry of the phosphorescent emitter. The coordinating ligands are described as cyclometalating (HC^N, eg. ppy) or ancillary (L or X^Y, eg. acac). They dictate color purity by modulating the energy levels and spatial geometry.
Electron-withdrawing substituents like fluorine increases the electron affinity of the complex and therefore the LUMO reduces in energy. Heteroleptic (with different ligands) transition metal complexes allow for the precise tuning of electronic and physical properties. The energy levels within a molecule can be adjusted to achieve the desired color emission by using ligands with electron-withdrawing or electron-donating groups and varying degrees of π-conjugation.
- Small Organic Molecules:
Small organic molecules, mostly made up of conjugated π systems, are typically host materials in PhOLEDs. They are responsible for reducing emission quenching processes such as triplet-triplet annihilation and exciton diffusion. The organic host is carefully selected based on their energy level alignment, charge transport properties and triplet energy. This maximizes the transition of the energy from the host to the guest emitter.
The molecular structure of host materials is also responsible for thermal and morphological stabilities. Through the manipulation of intermolecular interactions (via crystallization and rigidification) and host-guest assembly, non-radiative transitions in the emitter can be limited. Heteroatoms contribute to high triplet energy levels in host materials. This ensures that energy transfer to the dopant is efficient and prevents reverse energy transfer.
Advantages of Phosphorescent Organic Light-Emitting Diodes
The key advantage of phosphorescent organic light-emitting diodes is that they have internal electroluminescence quantum efficiencies of ~100%. Traditional fluorescent, singlet-emitting chromophore based OLEDs have a 3:1 ratio of non-emissive to emissive states. This severely limits device efficiency and has much greater energy demands. Phosphorescent emitters give access to triplet state emission even at room temperature.
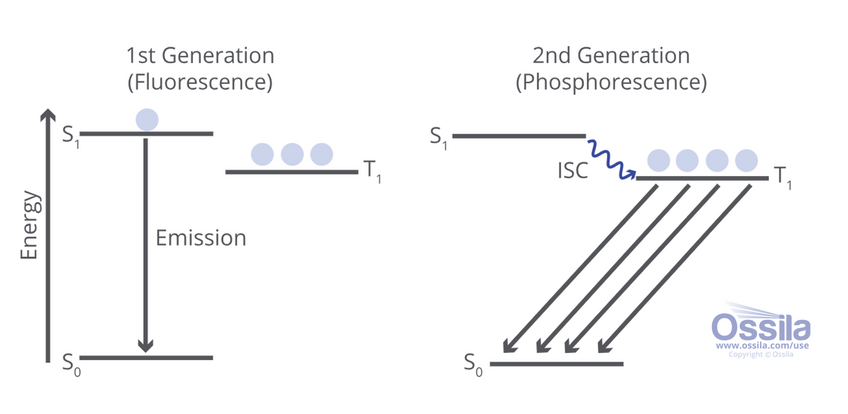
Phosphorescent materials not only increase the efficiency of OLEDs, they also have advantages including:
- Wide color range - through the selection of the dopant material different color light emission can be accessed
- High brightness and intensity - can be achieved using less energy due to higher efficiencies
- Flexibility in design - molecular engineering of organic components to control properties
- Thin and lightweight - compared to inorganic materials
- Customizable emission profiles - tuned by altering the chemical structure of host and dopant materials
Issues with Phosphorescent Organic Light-Emitting Diodes
Whilst phosphorescent organic light-emitting diodes are far more efficient that early generation OLEDs, they still face some key issues. In the quest for ever improved energy efficiency phosphorescent materials face issues including:
- Expensive and precious metals (IR, Eu, Pt) used in dopant materials .
- Quenching processes need to be addressed, including:
- triplet-triplet annihilation (TTA)
- energy transfer to charged molecules (polarons)
- dissociation of excitons into free charge carriers
- Complicated structures - require complex, multilayer architectures to optimize charge injection, exciton generation, and energy transfer.
- Degradation and lifetime issues - due to exciton-induced molecular instability, especially in high energy blue emitters.
- Efficiency roll-off - device's luminous efficiency decreases as current density increases.
Addressing these challenges requires the development of cost-effective dopant materials, advanced host-dopant systems to minimize quenching, and stable blue emitters. Innovations in device architecture and strategies to mitigate efficiency roll-off are critical for achieving long-term performance improvements in PhOLEDs.
OLED Materials
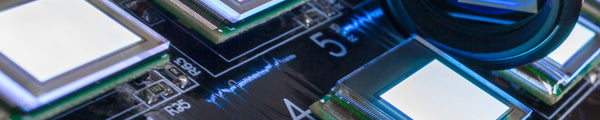
Read More...
Hyperfluorescence organic light-emitting diodes (HF-OLEDs) represent the 4th generation of OLED technology. Find out more.
Read more...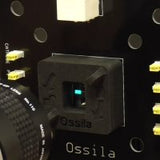
This guide gives you an overview of what to consider when characterising an OLED, as well as tips for their measurement.
Read more...
References
- Phosphorescent Materials, Huo, S. et al., ACS in Focus (2023)
- Excited-State Engineering in Heteroleptic Ionic Iridium(III) Complexes, Monti, F. et al., Accounts of Chemical Research (2021)
- Recent advances of donor-acceptor type carbazole-based molecules for..., Ledwon, P., Organic Electronics (2019)
- Ancillary ligand increases the efficiency of heteroleptic Ir-based..., Baek, S-Y. et al., Nature Communications (2020)
- Recent advances in organic luminescent materials with narrowband..., Ha, J. M. et al., NPG Asia Materials (2021)
Contributors
Written by
Application Scientist
Diagrams by
Graphic Designer