Application of Non-Fullerene Acceptors in Organic Solar Cells
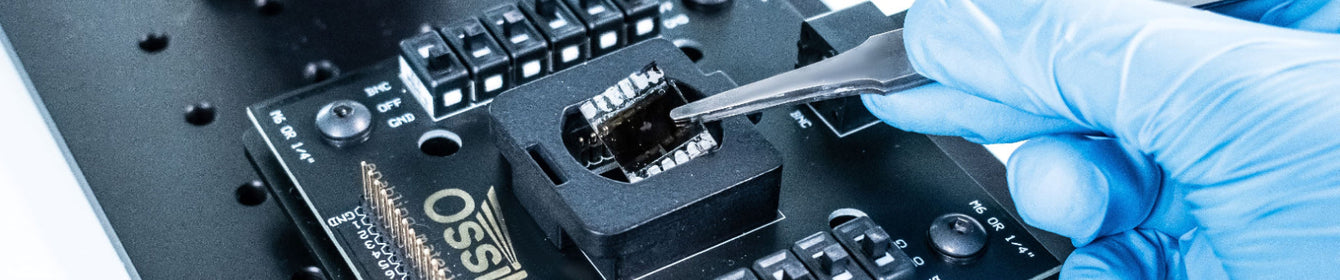
Non-fullerene acceptor (NFA) molecules are currently being used in the active layer of organic solar cells to enhance their efficiency. Organic solar cells, also known as organic photovoltaics (OPVs), consist of several organic components, each playing a distinct role in capturing solar energy. NFAs serve as electron acceptors and often absorb light as well, contributing to higher OPV power conversion efficiencies. They have been developed as alternatives to the earlier organic acceptors, fullerenes, which have reached their efficiency limits.
Typically organic solar cells that use NFAs are described as having bulk-heterojunction active layers. A blend of non-fullerene acceptor (such as Y6) and donor material (usually a polymer like PBDB-T-2F (PM6)) is used as the active layer, much like in polymer-fullerene bulk-heterojunction solar cells.
Advantages of Non-Fullerene Acceptors in Organic Solar Cells
Non-fullerene acceptors (NFAs) have been used in organic solar cells, leading to improved efficiencies compared to their fullerene-based counterparts. Power conversion efficiencies approximately 2.5% to 18% higher than those of organic solar cells using fullerene acceptors have been achieved. These improved efficiencies result from several advantages that NFAs offer, including:
- Easily synthesised
- Active layer morphology can be modified by NFA molecular tuning
- Electronic properties of NFAs can be tuned by chemical modifications
- Optical band gap can be altered to increase the absorption range of the active layer
Structure and Characteristics of NFAs
Non-fullerene acceptors are organic molecules with conjugation and different functional groups which allow electronic and optical properties to be tuned. NFAs typically fall into two different categories:
- Small-molecule acceptors (SMAs)
- Polymeric acceptors
SMAs are the most commonly used NFAs for organic solar cells, in particular fused-ring electron acceptors (FREAs). Within such NFAs, molecular design has resulted in optimisation of device morphology and charge transport properties. Typically NFAs will contain areas of electron-donating and electron-withdrawing chemical groups. These groups influence highest occupied molecular orbital (HOMO) and lowest unoccupied molecular orbital (LUMO) of the molecules and thus the band gap.
Conjugation is Key
The conjugated nature of the molecules not only allows for the rapid transport of electrons but forces a planar structure. This allows for specific packing and strong intermolecular interactions. The morphology of the active layer that contains the NFA is extremely influential over device efficiency. Using chemical groups to tune the solubility and processibility of NFAs has allowed the optimisation of morphology in bulk-heterojunction solar cells.
A popular NFA for use in organic solar cells is Y6. See the diagram to the right which shows the extent of molecular engineering in order to maximise power conversion efficiency. The different components within the Y6 molecule introduce a variety of characteristics which all play a role in improving device efficiency:
- Electron-deficient units: introduces electron affinity, influences molecular packing, conjugated and can contain heteroatoms for charge transport.
- Electron-rich units: supports charge carrier mobility, introduces large dipole moment (if asymmetric) to increase intermolecular interactions and influence packing.
- Side chains: influences solubility of the molecule and can be bulky to prevent too much aggregation of molecules, can influence the radiative recombination pathway.
- Bridging atoms: Heteroatoms here can provide electron-donating character, increase charge carrier mobility and increase the HOMO energy level.
-
π spacer: influences the extent of conjugation in the molecule which in turn impacts the electron donating ability of the backbone.
The same molecular engineering techniques have been used across different NFA families. Molecules based on the first NFA to be used in OPVs, ITIC, also have electron withdrawing substituents, a central fused-ring system and rigid side chains.
Types of Non-Fullerene Acceptors used in Organic Solar Cells
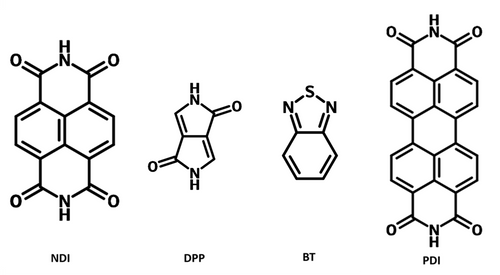
Non-fullerene acceptors (NFAs) are organic molecules that function as electron acceptors like fullerenes. However, unlike fullerenes, NFAs lack the hollow cage structure, providing greater flexibility in molecular design and enabling tunable electronic and optical properties. The most commonly used NFAs are built on highly conjugated structures such as:
- Indacenodithienothiophene (IDTT)
- Benzothiadiazole (BT)
- Naphthalene diimide (NDI)
- Perylene diimide (PDI)
- Diketopyrrolopyrrole (DPP)
A key breakthrough in NFA development was the creation of fused-ring electron acceptors (FREAs) like ITIC, which marked the beginning of a new era for non-fullerene acceptors in organic photovoltaics (OPVs). Since then, NFAs have revolutionized OPVs, with Y6, another fused-ring electron acceptor, becoming a focal point of study due to its high performance. NFAs now dominate bulk-heterojunction solar cell research due to their enhanced tunability, stability, and superior performance compared to fullerene-based molecules.
Indacenodithienothiophene Based Non-Fullerene Acceptors
Indacenodithieno[3,2-b]thiophene (IDTT) is a fused rigid highly conjugated structure of thienothiophene and s-indacene. The seven-ring-fused dihydrodithieno[2,3-d:2′,3′-d′]-s-indaceno[1,2-b:5,6-b′] dithiophene (IT) framework stands out as one of core building blocks for high-performance n-type molecules. IDTT is electron rich and the core structure of the ITIC-series non-fullerene acceptors.
ITIC represents the very first of the highly efficient NFAs achieving power conversion efficiencies rivalling those based on fullerenes acceptors. It has a IDTT core end-capped with two electron-deficient 2-(3-oxo-2,3-dihydroinden-1-ylidene)malononitrile (INCN) groups. The push–pull effect generated by the electron-rich IDTT core and electron deficient INCN end groups of ITIC facilitates intramolecular charge transfer (ICT) along the backbone of the structure, resulting in a broad and strong absorption of ITIC in the visible to near infrared region of 500–800 nm, peaking at 702 nm. ITIC, as a new class of acceptors developed for organic solar cells, overcomes some of the shortcomings of fullerene acceptors with weak absorption in the visible spectral region and limited energy-level variability.
Since the discovery of ITIC, further molecular engineering has improved absorption coefficients and shifted absorption wavelengths to enhance intramolecular electron transfer. The HOMO and LUMO energy levels have been tuned via the introduction of heteroatoms such as fluorine (eg. ITIC-4F). Through altering the electron-withdrawing/donating end groups the push-pull effect can be tuned. These properties makes it a better match for most polymer semiconductor donors and provides better stability for device fabrication and overall performance.
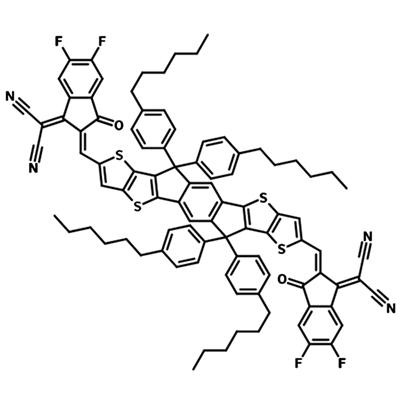
Benzothiadiazole Based Non-Fullerene Acceptors

The bicyclic 2,1,3-benzothiadiazole (BT) molecule consists of a benzene ring fused to a 1,2,5-thiadiazole ring. BT heterocyclic derivatives exhibit strong electron-withdrawing capabilities and are efficient fluorophores. Benzothiadiazole compounds are highly polarized, leading to intermolecular interactions such as heteroatom contacts and π–π stacking. These interactions are believed to contribute to their well-ordered crystal structures. Benzothiadiazoles possess a relatively high reduction potential and strong electron affinity, making them excellent material candidates for a variety of organic electronic devices.
As one of the most widely used electron-withdrawing units, benzothiadiazole plays an important role in the design of the non-fullerene acceptors, as well as semiconducting polymer and small-molecule donors. With four active sites from the benzene ring, BT offer rich variety of NFAs which could either be single, double, multiple benzothiadiazole-based, or benzothiadiazole fused pyrrole, thiophene or thienothiophene. A typical example of fused benzothiadiazole structure is thienothienopyrrole fused benzothiadiazole, the core structure of classic non-fullerene acceptor Y6.
Di(thienothienopyrrolo)-Benzothiadiazole Based Non-Fullerene Acceptors
The dithienothiophen[3.2-b]-pyrrolobenzothiadiazole based fused-ring system 12,13-dihydrodi(thieno[2′′,3′′:4′,5′]thieno[2′,3′:4,5]pyrrolo[3,2-e:2′,3′-g])benzothiadiazole. It is referred to as the backbone of Y6-series NFAs, another outstanding core unit for non-fullerene n-type molecules. Unlike the indacenodithienothiophene based NFAs, the Y6-series NFAs adopt an electron-deficient polycyclic arene as the core unit, in which one benzothiadiazole and two thienothiophene[3,2-b]-pyrrole units are fused to form a D-A-D type core structure. With two more electron-deficient end-groups, i.e. 2-(5,6-difluoro-3-oxo-2,3-dihydro-1H-inden-1-ylidene)malononitrile linked to the di(thienothienopyrrolo)-benzothiadiazole core by the π -linkers on both sides. This gives the complete skeleton structure of Y6-series NFAs, A-D-A0-D-A, alternating electron donating and accepting units.
The Y6-series NFAs exhibit a crescent shape and an asymmetric structure, showing a smaller Eg (energy gap) of 1.2 – 1.4 eV with the absorption of Y6-series NFAs extending to further infrared spectrum of 900–1100 nm through a strong ICT effect. Also, the alkyl side chains have been employed to control the intermolecular packing and solubility, prevent the rotation of the end-groups thereby lowering the energetic disorders to improve the charge transport. The end-group of Y6-series NFAs has largely been modified through chemical substitutions using electronegative atoms (e.g., F and Cl) to control the LUMO levels and molecular packing.
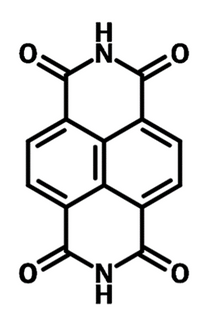
Naphthalene Diimide Based Non-Fullerene Acceptors
Naphthalene diimides (NDIs) are among the most investigated classes of organic semiconductors. Their optical and electronic properties can be modulated through functionalization with different chemical groups. The aromatic naphthalene core exists as a planar aromatic scaffold and two electron-withdrawing imide groups within the cyclic structure. As a result, naphthalene diimide possesses strong polarization of π conjugated system and low π electron density. This electron deficiency means NDIs show excellent electronic transfer ability. This property along with their stability in air make them suitable n-type semiconductors.
As well as excellent and tunable electronic properties, the optical properties of NDIs are attractive for application in organic solar cells. NDI normally shows an absorption band with an optical bandgap of 3 eV and broad absorption bands over the whole visible spectral range through the introduction of core substituents. One of the main strategies to narrow the bandgap is to introduce electron-donating groups. This induces intramolecular charge-transfer (ICT) from the peripheral unit to the NDI core.
The core unit itself can also be modified to extend the planar rigid core. For example, linking two NDI monomers via a vinyl group produces the dimer of N, N'-di(2-ethylhexyl)-2-bromonaphthalene-1,4,5,8-bis(dicarboximide) (BiNDI). This extends the π -conjugation length, planarizing the molecular backbone and enhancing the intermolecular π – π stacking.
Perylene Diimide Based Non-Fullerene Acceptors
Perylene-3,4,9,10-tetracarboxylic acid diimides (PDIs) and their derivatives have been one of the most promising classes of electron accepting materials. This is due to their high electron mobility, high molar absorption ecoefficiency, and exceptional chemical, thermal, and photochemical stability properties. PDI derivatives, exhibit broad optical absorption in the visible to near-infrared spectral region and show fluorescence with quantum yields near unity. Their strong electron-accepting character and significant charge transport properties in particular make them useful within organic solar cell devices.
The structure of PDIs include a rigid planar fused aromatic core that favours π–π intermolecular interactions. This leads to excellent structural stability, strong electron-accepting ability and high electron mobility (> 1 cm2 V-1 s-1) in their functional materials with n-type semiconducting characteristics. The chemical modification of the PDI structure at the nitrogen, bay and headland positions allows for the tuning of solubility, electronic and optical properties:
- Through forming PDI clusters via linkage at the nitrogen results in the destruction self-aggregation and an inhibition of intermolecular packing. This can lead to enhanced solubility and better film morphology.
- Modification at the bay position increases the conjugation length and improves the absorption properties of the molecule, thus alternating the molecular energy level and enabling better light-harvesting capabilities. This broadens the absorption spectrum into the visible and near-infrared regions, increasing the photocurrent generation and overall efficiency of the solar cell by capturing more sunlight across a wider range of wavelengths.
- Chemical modification at the headland positions can enhance π – π stacking to improve the electron mobility of the molecule. Additionally, improved π–π stacking can contribute to the thermal and photochemical stability of the material, further extending the device's operational lifetime.
Diketopyrrolopyrrole Based Non-Fullerene Acceptors
Diketopyrrolopyrrole (DPP) bicyclic core contains two secondary amine units and two carbonyl groups. The molecule has excellent electron-withdrawing capacity and charge transfer properties. These properties make the DPP core perfect for donor-acceptor (D-A) type conjugated materials.
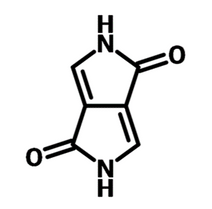
DPP-based materials exhibit:
- strong π-π intermolecular interaction
- aggregation
- good planarity
- good charge carrier mobility
- elevated thermal stability
- remarkable absorbance in the UV–visible and near-infrared range
DPP chromophore and its derivatives have played a key role in the molecular design and construction of high-performance materials in electronic devices, including sensors, OFETs and OSCs.
Pyrrole-based NFAs, as the main kind of N-heteroarene based NFAs, exhibited great potential in fabricating high performance OSCs for the strong electron-donating nitrogen atom, facilitating higher energy levels, red-shifted absorption and being an extra reaction site for side chain modification.
Research and Development of NFAs in Organic Solar Cells
As NFA-based organic solar cells have reached efficiencies that vastly surpass those achieved using fullerenes, the expansion of the range in molecules has grown rapidly. There have been developments in NFAs based on a variety of conjugated cores. There are too many to cover on this page but the table below shows a variety of examples of NFAs used in organic solar cells, their properties and their impact on device performance:
NFA Core / Family | NFA | NFA Properties | Polymer Donor | Organic Solar Cell Device Performance | Ref |
---|---|---|---|---|---|
IDTT | ITIC |
|
PBDB-T |
Max Power Conversion Efficiency (PCE) of 11.21 % VOC of 0.90V JSC of 16.73 mA cm−2 FF of 70.8% Certified Efficiency of 10.78 |
|
IDTT | ITIC-4F |
|
PBDB-TF |
PCE of 13.7% FF of 75% JSC of 20.39 mA cm−2 |
|
BT | Y6 |
|
PBDB-TF |
PCE of 18.22 % Certified efficiency of 17.6 % |
|
BT | BTP-eC9 |
|
PBDB-TF | PCE of 17.8 % | |
BT |
(Some L8-ThCl) |
|
D18 | PCE of 19.4 % | |
BT |
(Some L8-BO) |
|
D18 | PCE of 20.1 % | |
BT | BFC-4F |
|
PTQ10 |
PCE of 12.05 % VOC of 0.81 V Enhanced JSC of 20.50 mA cm-2 |
|
NDI | BiNDI |
|
PTB7 | PCE of 2.41 % | |
PDI | FTTB-PDI4 |
|
P3TEA | PCE of 10.58 % | |
DPP | SF(DPPB)4 |
|
P3HT |
PCE of 5.16 % VOC of 1.14 V |
Non-Fullerene Acceptors Mentioned
Challenges and Limitations of NFAs in Organic Solar Cells
Non-fullerene acceptors (NFAs) have transformed organic photovoltaics (OPVs) by boosting power conversion efficiencies (PCEs) and overcoming limitations of fullerene-based systems. However, despite this, NFAs face several key challenges and limitations that must be overcome for their widespread adoption and commercialization in real-world applications:
- Improving the device lifetime under real-world conditions: One of the major hurdles for NFAs is ensuring long-term stability. Many NFAs degrade when exposed to environmental factors such as oxygen, moisture, and ultraviolet (UV) light. These factors can lead to the deterioration of active layers, reducing the performance and overall lifespan of solar cells. Most NFAs must be handled in controlled environments such as glove boxes, especially during device fabrication. This is not ideal for large-scale production. Addressing this issue requires both the development of more robust materials and the improvement of encapsulation techniques that can protect NFAs from environmental degradation. Importantly, without compromising on device efficiency.
- Enhancing large-scale manufacturability: While NFAs have demonstrated impressive lab-scale performance, scaling up the production of organic solar cells (OSCs) remains challenging. The process of fabricating NFA-based solar cells often involves complex and precise deposition techniques. Many of which are not be suitable for high-throughput, cost-effective manufacturing. Additionally, ensuring uniform film formation over large areas without compromising the material's electronic properties is crucial. Researchers are exploring methods such as roll-to-roll printing and solution processing, but significant work is needed to streamline these techniques for large-scale manufacturing.
- Molecular tuning and device architecture integration: Ongoing research into fine-tuning the molecular structures of NFAs aims to enhance their optical, electrical, and morphological properties. By optimizing energy levels, charge mobility, and solubility, scientists aim to further improve the performance and stability of NFA-based devices. NFAs are also being integrated into advanced device architectures, such as tandem solar cells, where they can contribute to broader absorption spectra and higher overall efficiencies. Despite this potential, optimizing the compatibility between NFAs and different layers in complex device architectures remains a technical challenge.
Non-Fullerene Acceptors
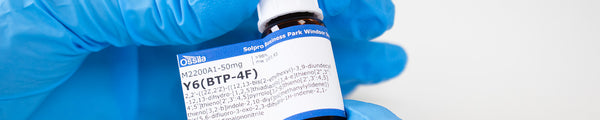
Learn More
References
- Recent progress in organic solar cells based on..., Luo, D., J. Mater. Chem. A (2022)
- Single-Junction Organic Solar Cell with over 15% Efficiency..., Yuan, J., Joule (2019)
- Enabling low voltage losses and high photocurrent in..., Yuan, J., Nature Communications (2019)
- Vinylene π-bridge: A simple building block for ultra-narrow..., Hai, J., Dyes and Pigments (2021)
- Fullerene-Free Polymer Solar Cells with over 11% Efficiency..., Zhao, W., Advanced Materials (2016)