Fluorescence and Phosphorescence
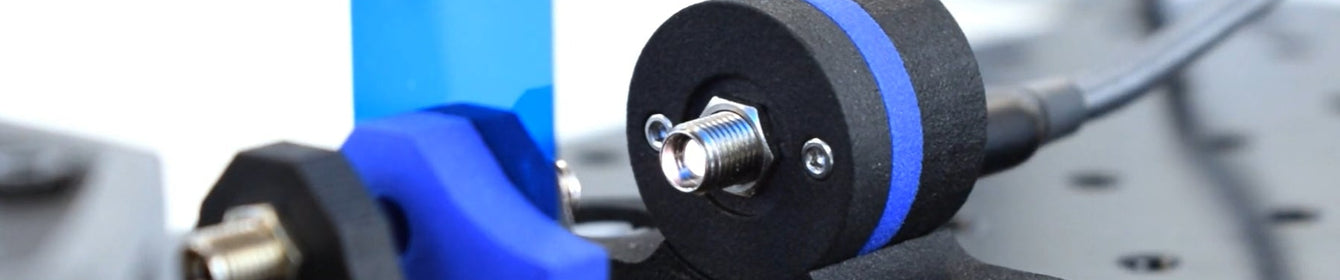
The main difference between fluorescence and phosphorescence is the time between absorbance and the emission of photons:
- When fluorescent materials, such as Alq3, absorb a photon, they almost immediately emits a lower energy photon.
- When phosphorescent materials, like Ir(ppy)3, absorb a photon, there is a delay before it is remitted.This is because requires a forbidden transition.
Fluorescence and phosphorescence are both types of photoluminescence. These are types of radiative emission so after a photon is absorbed, a lower energy photon is emitted as the electron relaxes back to the ground state.
On an atomic level, the distinction between phosphorescence and fluorescence depends on the electron spin state during energy level transitions. In fluorescence transitions, this electron spin state is maintained throughout. Phosphorescence, however, requires the spin state to change when a photon is absorbed. This is reversed when the subsequent emission occurs. Another key difference is that fluorescence only occurs when light is incident on the material, while phosphorescent materials can continue to glow sometime after the light source has been removed.
Fluorescent materials can be used for many different purposes. These include bioluminescent technology, investigating optical properties of materials, or labelling molecules in medicine, microbiology, or chemistry. On the other hand, the long emission life, high-signal-to-nose ratio, large stokes shift and a higher quantum yield of phosphorescent materials make them especially attractive in many fields. Phosphorescent materials are especially useful for tracking biological processes and chemical reactions, for emergency signage and other applications requiring long lifetime emission.
Singlet and Triplet States
In its ground state, the total spin angular momentum of a molecule will be S=0. This is because all electronic orbitals are full (two electrons) and the electron spins in each orbital are anti-parallel (opposite direction). This is known as a singlet state. However, when an electron from the HOMO is excited to the LUMO, there are then two unpaired electrons, and the total spin angular momentum can be either S=0 or S=1. S=0 states are called singlets and S=1 states are called triplets. This is because the multiplicity of the spin states (the number of degenerate states) is given by 2S+1.
- When the excited electron retains its spin from the ground state (i.e. they are anti-parallel), S=0 and the multiplicity = 2(1/2 + -1/2) + 1 = 2(0) + 1 = 1. Hence, singlet states.
- When the excited state electron changes spin state so it's the same as the ground state (parallel), S=1 and the multiplicity = 2(1/2 + 1/2) + 1 = 2(1) + 1 = 3. Hence, triplet states.
According to Hund's rule of maximum multiplicity, the lowest energy state will be the one in which the multiplicity is largest. This means that electrons will fill orbitals singly and with parallel spins before pairing up. Triplets therefore tend to have a slightly lower energy than singlets.
Fluorescence Transitions
Fluorescence consists of only spin-allowed transitions. As photons have spin-0, only transitions between states of the same spin, i.e. singlet-singlet, are spin-allowed. Therefore, where S0 is the singlet ground state (or highest occupied molecular orbit), and S1 is the singlet excited state (or lowest occupied molecular orbit), the absorption edge will tend to correspond to the S0 → S1 transition. Similarly, the emission peak will also tend to correspond to the S1 → S0 transition.
The emission of a photon when an electron relaxes from the S1 → S0 state is known as fluorescence and has a very short lifetime (~ns).
Examples of Fluorescent Molecules
Phosphorescence Transitions
Just like for fluorescence emissions, in phosphorescence, an electron is initially excited through absorbance of a photon. The electron can then non-radiatively relax into the triplet state through intersystem crossing (ISC). This transition is not spin-allowed but can occur due to spin-orbit coupling. Once the electron is in the triplet state, it will eventually radiatively relax into the ground state (S0) or one of the vibrational states above. Although singlet-triplet transitions are forbidden by spin selection rules, they can still occur over a longer time period. This type of emission is termed phosphorescence and can have lifetimes of seconds or even as long as several hours.
Examples of Phosphorescent Molecules
Read More...
References
- T.-S. Ahn, et al. Rev. Sci. Instrum. 78, 086105 (2007).
Contributing Authors
Written by
PhD Student Collaborator
Reviewed by
Product Specialist
Application Scientist