Fullerene vs Non-Fullerene Acceptors for OPVs
Fullerene and non-fullerene acceptors play a pivotal role in organic photovoltaics (OPVs). OPVs are promising candidates for next-generation solar cells due to their lightweight, flexibility, and potential for low-cost production. The acceptor materials are crucial for determining the efficiency of charge separation, transport, and ultimately the overall performance of the solar cells. Fullerene-based acceptors have long been the standard, but non-fullerene acceptors have recently received significant attention due to their potential to overcome some of the limitations associated with fullerene-based materials.
The Differences Between Fullerene and Non-Fullerene Acceptors
Here we explore the differences between fullerene and non-fullerene acceptors, comparing their chemical structure, electronic properties, photophysical behaviour, charge transport, morphology, stability, and potential for large-scale application. By examining these factors, we can understand why the shift toward non-fullerene acceptors (NFAs) is emerging as a key trend in the field of organic photovoltaics.
Feature | Fullerene | Non-Fullerene Acceptor |
---|---|---|
Chemical Structure | Simple, not very tunable | Very tunable |
Electronic Properties |
Excellent electron-accepting properties HOMO and LUMO not easily tuned |
Excellent electron-accepting properties HOMO and LUMO easily tuned |
Photophysical Behaviour | Relatively weak absorption in the visible spectrum | Can absorb a broader range of wavelengths |
Charge Transport |
Relatively good electron transport properties Balanced charge mobility Poor hole-blocking properties High non-radiative recombination losses |
Efficient charge transport pathways Reduced recombination losses Initially lower electron mobilities than fullerenes but can be tuned to increase efficiencies Better energy level alignment |
Morphology | Issues of aggregation and phase separation due to spherical shape of fullerenes |
Better control over morphology Planar nature of molecules improves packing Functionalization with different groups to improve processibility |
Stability | Prone to photochemical degradation |
Molecules often need handling in a glovebox Once in a device more resistant to photodegradation and thermal stresses aggregation than fullerenes |
Scalability | Complicated production methods | Simpler and more versatile synthesis routes |
Chemical Structure
Fullerenes are hollow spherical carbon molecules. C60 and C70 and the most common, and their derivatives, such as [6,6]-phenyl-C61-butyric acid methyl ester (PCBM) and its analogs with solublising functional groups. The basic structure of fullerenes consists of 60 or 70 carbon atoms arranged in a truncated icosahedron, which resembles a soccer ball. This highly symmetric structure is a defining characteristic of fullerenes and contributes to their electron-accepting properties. PCBM, for example, is a widely used fullerene derivative that improves solubility in organic solvents, enabling easier processing in OPVs.
In contrast, non-fullerene acceptors (NFAs) are based on a wide variety of molecular structures, often designed to have more flexibility and tunability compared to fullerenes. NFAs are usually conjugated organic molecules that incorporate electron-withdrawing units (such as diketopyrrolopyrrole (DPP), naphthalene diimide (NDI), and perylene diimide (PDI)) and electron-donating units in a donor-acceptor (D-A) configuration. This modularity allows researchers to fine-tune the optoelectronic properties of NFAs. Some popular NFA structures include indacenodithieno[3,2-b]thiophene core based ITIC-series (IT-4F), Y-series molecules (Y6), and a wide range of A-D-A type small molecules. The primary structural difference is that NFAs allow for a far greater diversity of molecular architectures, while fullerene acceptors are constrained by the rigid, spherical structure of C60 and C70.
Electronic Properties
Fullerenes, due to their high electron affinity and three-dimensional conjugation, exhibit excellent electron-accepting properties. Their LUMO energy levels are generally deep, facilitating efficient electron transfer from the donor material in OPVs. However, their limited tunability in terms of energy levels is a significant drawback. Fullerene acceptors typically have high LUMO levels, which limits their ability to fine-tune the open-circuit voltage (VOC) of solar cells. Moreover, the strong electron affinity of fullerenes makes it challenging to precisely control the energy gap between the donor and acceptor materials, leading to relatively lower VOC values.
Non-fullerene acceptors offer far more flexibility in tuning electronic properties. By varying the donor and acceptor units in the molecular structure, the energy levels LUMO and HOMO can be precisely controlled. This allows NFAs to achieve a better match with donor materials, leading to higher VOC values and improved overall device efficiency. NFAs have a wider range of LUMO levels, which can be designed to optimize charge separation and energy alignment with donor materials. This tunability enables better control of both VOC and the short-circuit current (JSC), leading to higher power conversion efficiencies (PCEs).
Photophysical Behaviour
Fullerenes possess excellent electron mobility and tend to form well-ordered, crystalline domains, which are beneficial for charge transport. However, they have relatively weak absorption in the visible spectrum, which limits their contribution to light absorption in OPV devices. As a result, the donor material in fullerene-based OPVs is often responsible for absorbing most of the incident light. Furthermore, fullerene acceptors suffer from high rates of recombination and poor exciton dissociation, as their spherical structure limits the formation of pure donor-acceptor interfaces.
Non-fullerene acceptors, on the other hand, can be designed to absorb a broader range of wavelengths, including more of the visible and near-infrared spectrum. This results in better photon harvesting and, consequently, higher current generation. Fullerenes, on the other hand, have limited absorption beyond 500 nm, which restricts the amount of sunlight that can be utilized by the solar cell. NFAs can be designed with strong absorption in the 600-900 nm range, leading to improved external quantum efficiencies (EQEs). This enhanced light absorption can increase JSC and overall device efficiency. In addition, NFAs generally exhibit lower recombination rates and better exciton dissociation efficiencies. This can be attributed to their planar or semi-planar structures, which allow for better donor-acceptor interface formation, leading to more efficient charge generation and transport.
Charge Transport
Fullerenes have high electron mobility, a critical factor in ensuring efficient charge transport within the OPV device. The spherical shape of fullerenes allows for isotropic charge transport, meaning that electrons can move in multiple directions within the fullerene domains. This results in relatively good electron transport properties and balanced charge mobility. However, fullerenes also exhibit relatively poor hole-blocking properties, which can lead to charge recombination and lower overall device efficiency. One of the main issues with fullerene-based OPVs is the relatively high non-radiative recombination losses, which lower the efficiency of the device.
NFAs are often designed with a planar or semi-planar structure that promotes better packing and crystallinity in thin films. This leads to more efficient charge transport pathways and reduced recombination losses. While NFAs may initially have lower electron mobility than fullerenes, the increased tunability of molecular packing and morphology allows researchers to optimize NFAs for balanced electron and hole transport, improving the overall efficiency of OPVs. Additionally, NFAs can be designed to have better charge-carrier mobility through modifications in their conjugation length and molecular stacking, which further enhances charge extraction and reduces losses. Moreover, NFAs exhibit lower non-radiative energy losses due to better energy level alignment and molecular design strategies, leading to improved power conversion efficiency (PCE).
Morphology and Film Formation
The spherical nature of fullerene molecules often leads to poor film formation and undesirable phase separation in blend films. Fullerenes tend to aggregate and form large crystalline domains, which can disrupt the donor-acceptor interface and hinder efficient charge separation. Additionally, fullerenes can phase-separate from the donor material over time, leading to device degradation.
Non-fullerene acceptors, however, offer better control over morphology compared to fullerene-based acceptors. OPV cells are known to suffer from morphological degradation over time, especially when exposed to heat and light. The planar structures of NFAs allow for improved molecular packing and the formation of more stable donor-acceptor interfaces. This results in finer phase separation, which is crucial for efficient exciton dissociation and charge transport. NFAs also tend to exhibit better compatibility with a wide range of donor materials, leading to more stable and homogeneous blend films. This not only improves initial device performance but also enhances the long-term stability of OPVs.
Stability
One of the main drawbacks of fullerene acceptors is their poor stability under operational conditions. Fullerenes are prone to photochemical degradation, which leads to a decline in device performance over time. Additionally, fullerene-based OPVs tend to suffer from poor thermal stability, as fullerenes can aggregate under elevated temperatures, leading to phase separation and performance loss.
NFAs have shown significantly better stability compared to fullerenes when in a given OPV device. The more robust molecular structures of NFAs provide improved resistance to photodegradation and thermal stress. Furthermore, NFAs are less prone to aggregation, which enhances the thermal stability of the active layer and contributes to the long-term operational stability of the device. Recent studies have demonstrated that NFA-based OPVs can achieve lifetimes comparable to or even exceeding those of traditional silicon-based solar cells, making them more viable for large-scale commercial applications. However, NFAs are often highly sensitive before being incorporated into the device and require inert processing conditions.
Scalability and Cost
Fullerene acceptors, such as PCBM, have been commercially available for many years and are relatively easy to process. However, fullerenes are often synthesized through complex and costly processes, which can limit their scalability for large-scale production. The high cost of fullerene-based materials remains a significant barrier to the widespread adoption of fullerene-based OPVs.
Non-fullerene acceptors offer greater potential for cost reduction and scalability due to their simpler and more versatile synthesis routes, allowing for greater structural diversity. This opens a wider range of design possibilities, making NFAs highly customizable for different device architectures. Many NFAs can be synthesized from readily available organic building blocks, reducing material costs. Additionally, the increased flexibility in molecular design allows for the development of NFAs that are specifically tailored for solution processing and large-scale fabrication techniques, such as roll-to-roll printing. As NFA materials become more widely adopted, the cost of producing NFA-based OPVs is expected to decrease, making them more competitive with traditional silicon-based solar cells.
Fullerene and Non-Fullerene Mixture Acceptors
An organic solar cell with a ternary active layer is an effective strategy to enhance absorption and optimize morphology. The active layers comprise a combination of three donor and acceptor components. The introduction of a fullerene derivative, such as PCBM, has been shown to improve light absorption and the open-circuit voltage in polymer donor:NFA acceptor binary systems. In the ternary system, PCBM works as a morphology regulator to increase the contact interface between the polymer layer and NFA acceptor layer, facilitating the NFA diffusion into the polymer layer. The ternary system with both fullerene and non-fullerene mixture acceptors helps to enhance the dissociation of the excitons and provide a cascade energy level alignment for efficient charge transport.
One of the great advantages of ternary systems while compared to binary OSCs is that the third component can offer complimentary absorption of the light spectrum thus improve the photon absorption. Apart from being able to absorb light more efficiently with the introduction of the third component, the ternary system also offers appropriate phase separation by intermolecular interactions thus it improves the morphology of the active layers. The ternary system can also open new charge transport channels to direct the charge carriers to transport more effectively from the active layer to the electrode. Also, without complicating the device fabrication process, the third component can be easily made into the mixture of the binary system by simply processing the ternary system as a single layer. Finding the right recipe of the weight ratios of each component, the processing solvent, and sometime the appropriate regulating solvent or solid additives are key factors in promoting device performance.
In the ternary polymer donor:NFA:fullerene system, the LUMO energy (−3.9 eV) level of PCBM lies between the LUMO (−3.6 eV) of polymer donor, i.e. PBDB-TF (PM6) or PBDB-TCl (PM7), and the LUMO (−4.1 eV) of NFA acceptor IT-4F or Y6, resulting the increased Voc. After blending with PCBM, both the hole and electron mobilities are increased to reduce the charge recombination. With the addition of PCBM into the binary blend, the phase purity is increased while the homogenous film morphology and the π–π stacking patterns of the host binary structure are maintained. The enhanced charge mobility, the reduced charge recombination, the increased phase purity, as well as the complimentary absorption spectrum, are responsible for the improved fill-factor (FF) and increased short-circuit current (JSC) density.
Summary
The shift from fullerene to non-fullerene acceptors represents a major advancement in the field of organic photovoltaics. Fullerene acceptors, while historically important for the development of OPVs, suffer from limitations such as poor light absorption, limited tunability, and instability. Non-fullerene acceptors, by contrast, offer improved tunability, better light absorption, enhanced stability, and more efficient charge transport.
The ability to design NFAs with tailored electronic properties, better morphology control, and improved stability makes them a more promising candidate for the next generation of high-efficiency organic solar cells. With continued research and development, NFA-based OPVs have the potential to surpass the limitations of fullerene-based systems and become a leading technology in the renewable energy sector. Fullerene and NFA mixture active layers have also proven a great success in promoting power conversion efficiency in OPV device.
Non-fullerene acceptors
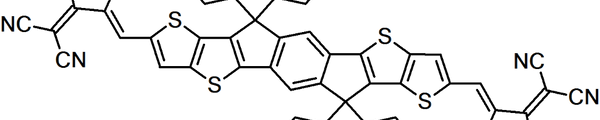
Learn More
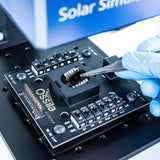
Non-fullerene acceptor (NFA) molecules are currently being used in the active layer of organic solar cells to enhance their efficiency. Organic solar cells, also known as organic photovoltaics (OPVs), consist of several organic components, each playing a distinct role in capturing solar energy.
Read more...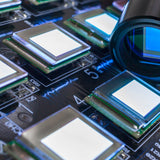
Organic solar cells, also known as photovoltaics (OPVs), have become widely recognized for their many promising qualities. This page introduces the topic of OPVs, how they work and their development.
Read more...Further Reading
- Zhao et al. (2016); Efficient organic solar cells processed from hydrocarbon solvents, Nat. Energy, 1, 15027; DOI: 10.1038/nenergy.2015.27.
- Li et al. (2018); Achieving over 11% power conversion efficiency in PffBT4T-2OD-based ternary polymer solar cells with enhanced open-circuit-voltage and suppressed charge recombination, Nano Energy, 44, 155-163; DOI: 10.1016/j.nanoen.2017.12.005.
- Speller et al. (2019); From Fullerene Acceptors to Non-Fullerene Acceptors: Prospects and Challenges in the Stability of Organic Solar Cells, J. Mater. Chem. A, 7, 23361-23377; DOI: 10.1039/C9TA05235F.
- Zhu et al. (2018); High-efficiency small-molecule ternary solar cells with a hierarchical morphology enabled by synergizing fullerene and non-fullerene acceptors, Nat. Energy, 3, 952–959; DOI: 10.1038/s41560-018-0234-9.