Molecular Design Principles of MR-TADF Materials
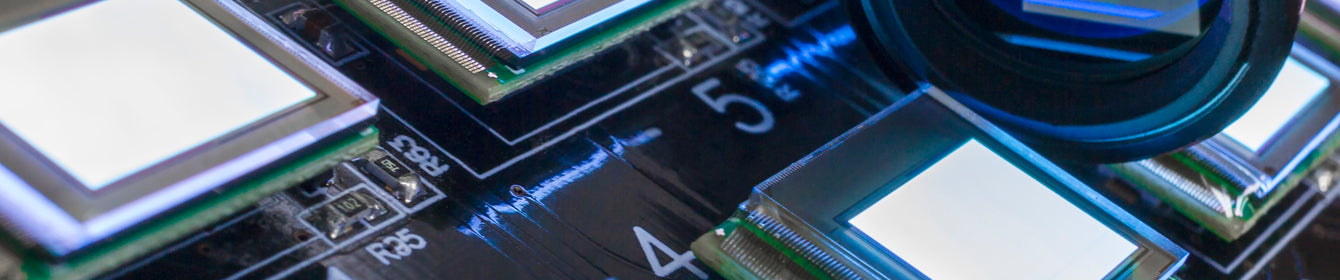
The design of multiple resonance thermally activated delayed fluorescent (MR-TADF) materials requires careful selection of molecular scaffolds and substituents to achieve the desired photophysical properties i.e. color and color purity. In general, the reversed intersystem crossing (RISC) process is considered the bottleneck for achieving highly efficient TADF. To enhance the RISC rate constant, it is key to have a small singlet-triplet energy difference (ΔEST) and large spin-orbit coupling (SOC).
There are two key requirements to consider in the molecular design of MR-TADF materials:
- A small ∆E(T1 → S1) (<200 meV) is required to induce T1 → S1 reverse intersystem crossing
- A large transition-dipole moment between S1 and ground-state S0 to accelerate the rate of S1 → S0
What are the Benefits of MR-TADF Molecular Design?
Traditional TADF materials can undergo geometrical deformation between the donor and acceptor units causing broad intramolecular charge-transfer emission with substantial Stokes shift. MR-TADF molecular design addresses both problems by atomic separation of frontier molecular orbitals via opposite resonance effect of electron-rich nitrogen and electron-deficient boron in a rigid molecular framework.
In MR-TADF materials, the electron donating and accepting atoms or groups are in close vicinity and the charge transfer in the relevant singlet and triplet excited states occurs between neighbouring atoms, an electron transfer process depicted as short-range charge transfer (SRCT). Gaining SRCT has the advantage of decreasing the ΔEST by minimizing the overlap between the involved excited states yet still maintaining some degree of overlap to not fully hinder the oscillator strength.
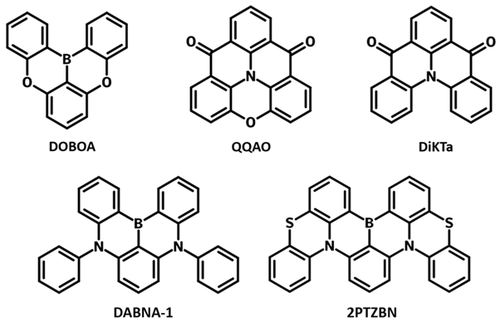
The atomic separation of frontier molecular orbitals via opposite resonance effect of neighbouring electron-rich and electron-deficient atoms in a rigid molecular framework also promises of compressed full width at half maximum (FWHM) of emission band with great color purity. Having electron donating atom and electron deficient atom disposed para to each other, MR-TADF is a new class of fused planar polycyclic aromatic compounds.
Example Core Structures
- DABNA-1 and DOBOA: the multi-resonance effect comes from a polycyclic skeleton containing boron and nitrogen atoms, and boron and oxygen atoms, respectively.
- DiKTa and OQAO: the MR effect comes from carbonyl groups and nitrogen atoms.
- 2PTZBN: the sulfur-incorporated structure, enables considerable rate constant of RISC (kRISC) up to 2.8 × 105 s−1 in toluene due to larger spin-orbital coupling (SOC) values and smaller singlet–triplet energy splitting (ΔEST). Consequently, organic light-emitting diodes based on 2PTZBN exhibited highly efficient green emission with maximum external quantum efficiency (EQE) of 25.5%.
Molecular Design Principles for MR-TADF Materials
Though nearly full-color emission has been accomplished for MR-TADF emitters, this class of materials has still known to exhibit poor kRISC values (~ 104 s,sup>–1) and severe efficiency roll-off at high current densities. To enhance the kRISC values while maintaining a reasonable large spin-orbit coupling, and to alleviate the efficiency roll-off in TADF-OLEDs, following strategies of molecular design have been adopted for MR-TADF materials:
Heavy Atom Effect
The inclusion of heavy atoms, such as selenium and sulfur, in MR-TADF molecular structures increases the spin orbit coupling (SOC) between singlet and triplet states. As a result, intersystem crossing and reverse intersystem crossing rates are faster. To minimize device efficiency roll-off, both singlet and triplet excitons should be quickly converted.
In MR-TADF materials, the fast radiative rate of singlet excitons is governed by the intrinsic large Frank-Condon overlap integral. Access to triplet excitons depends on the RISC channel. The rate of reversed intersystem crossing kRISC becomes the key factor to reduce the population of triplet excitons and realize low efficiency roll-off.
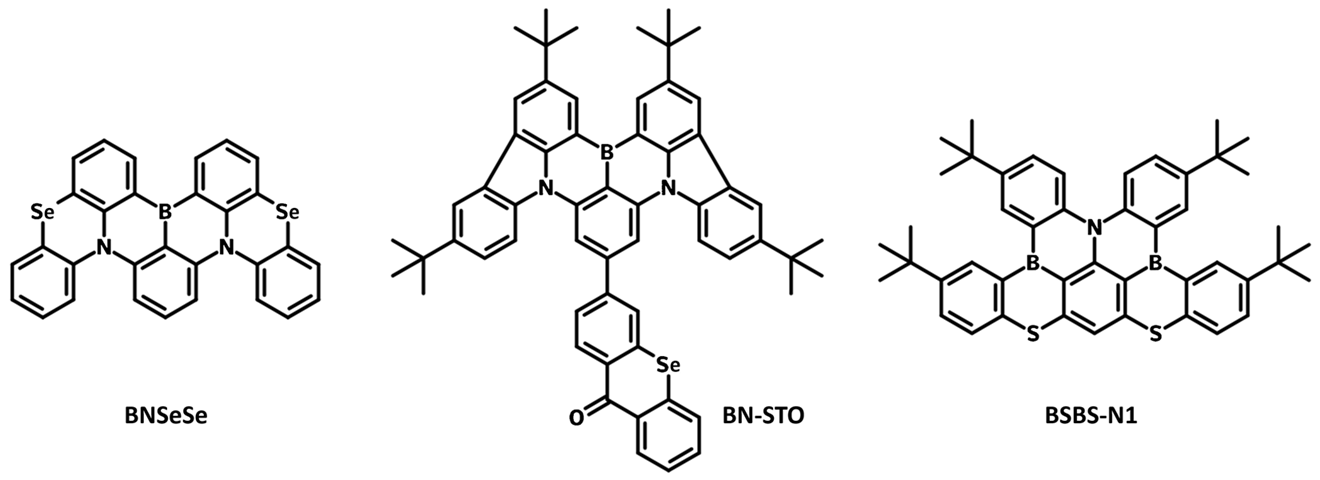
According to Fermi’s golden rule, the kRISC in TADF systems mainly depends on spin-orbit coupling (SOC) and energy splitting between S1 and T1 states. To enhance kRISC, one of the most practicable strategies is to narrow the ΔEST to provide a small energy gap channelling the up-conversion process from low-energy T1 to high-energy S1 state, boosted by thermal energy.
Examples of heavy atom molecular engineering
Molecule | Heavy Atom | Emission Peak / nm | FWHM / nm | PLQY / % | kRISC / s-1 | EQE / % | Ref |
---|---|---|---|---|---|---|---|
BNSeSe | Selenide | 514 | 38 | 100 | 2.0 × 106 | 36.8 | |
BN-STO | Selenide | 517 | 34 | - | 1.2 × 105 | 40.1 | |
BSBS-N1 | Sulfur | 478 | 24 | 89 | 1.9 × 106 | 21 |
|
Extended Charge Delocalization
The extension of the core π-skeleton is an effective strategy to achieve the simultaneous reduction of ΔEST, enhancement of oscillator strength, and retention of narrow line width in MR-TADF emitters. A prime example is the blue emitter ν-DABNA, featuring a fully resonating π-skeleton with 9 fused rings with alternating electron-accepting boron and electron-donating nitrogen atoms. Despite the extended conjugation, the design ensures a clear MR effect, with HOMO and LUMO localized on adjacent N and B atoms.
This structure minimizes bonding/anti-bonding interactions and vibronic transitions, resulting in ultra-narrow photoluminescent spectra. The extended conjugation enhances charge transfer, enabling ν-DABNA to achieve higher kRISC and quantum efficiency than its precursor DABNA-1. As a results it has an EQE of 34.4% and mitigated efficiency roll-off. Additionally, ν-DABNA demonstrates excellent performance as a deep blue emitter, achieving 100% PLQY and narrowband emission (FWHM: 14 nm) in toluene.
Examples of extended charge delocalization molecular engineering
Molecule | Emission Peak / nm | FWHM / nm | EQE / % | ΔEST | Ref |
---|---|---|---|---|---|
BCz-BN | 484 | 25 | 25.3 | 0.16 | |
NBO | 487 | 27 | 26.1 | 0.12 | |
NBNP | 500 | 29 | 28.0 | 0.09 |
By fusing carbazole or dibenzofuran into the boron-nitrogen skeleton, π-extended MR-TADF emitters NBO and NBNP are obtained, enhancing charge transfer delocalization and minimizing ΔEST. These emitters show red-shifted photoluminescence peaks, improved SOC values, and nearly 10x higher RISC rates than their parent BCz-BN. OLEDs based on NBO and NBNP achieve maximum EQEs of 26.1% and 28.0%, respectively, with low efficiency roll-offs.
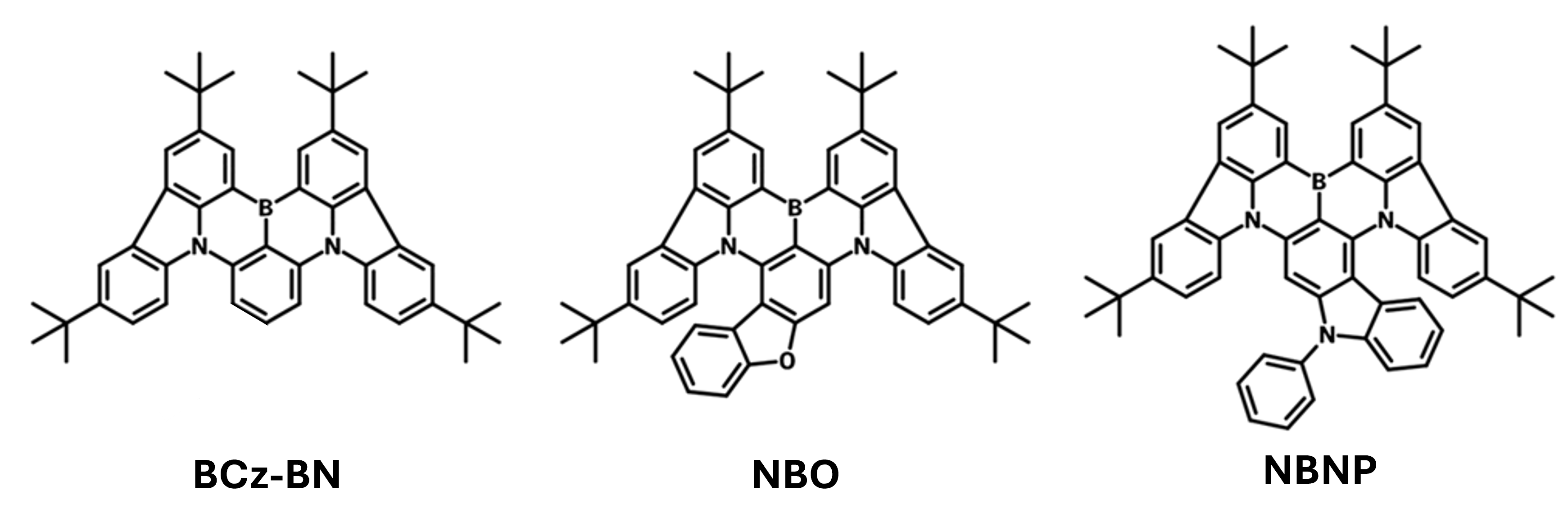
Nonplanar Skeletons or Bulky Substituents
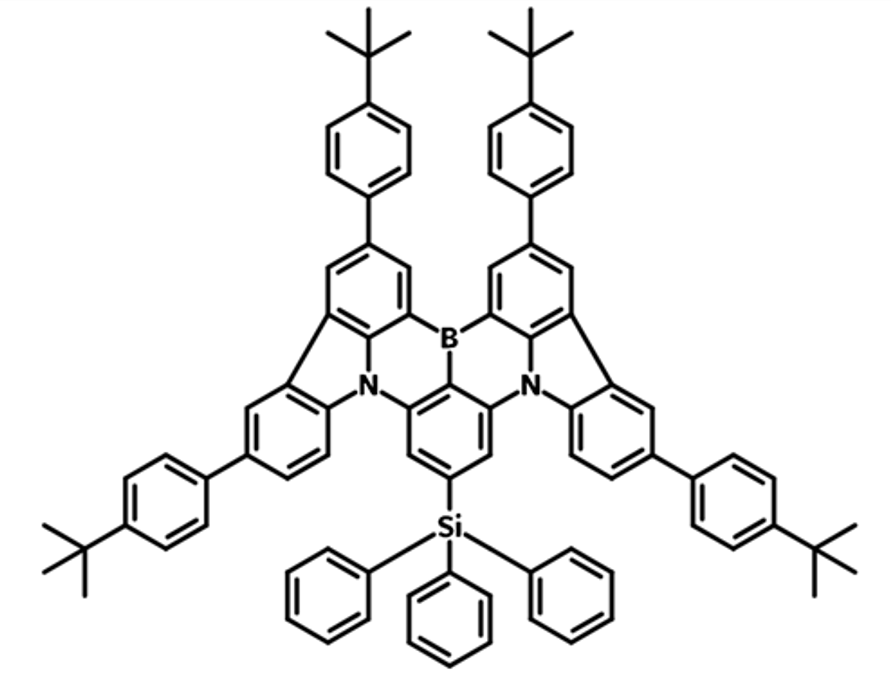
Introducing bulky substituents can induce steric effects and effectively weaken intermolecular forces. As a result the tendency of molecules to aggregate and form excimers is reduced. The prevention and reduction in aggregation can help mitigate concentration quenching and spectral broadening, which are common issues in solid state optoelectronics.
A key challenge for MR-TADF emitters is severe aggregation-caused quenching (ACQ) and spectral broadening in the solid state, resulting from intense π–π interactions. To mitigate the formation of less emissive aggregates or excimers, extremely low doping concentrations are required, which leads to broadened linewidths and suboptimal device performance. Developing MR-TADF emitters that are insensitive to doping concentration is crucial for achieving better color purity and improved device performance. Some examples of bulky substituents include:
- Carbazolyl units
- Twisted heptagonal tribenzo[b,d,f]azepine unit
- Nonplanar triphenyl silyl (Ph3Si-) units
- Tert-butylphenyl substituents
Examples of heavy atom molecular engineering
Molecule | Structure | Key Features | EQE / % | Ref |
---|---|---|---|---|
BN-CP1 | Planar BCz-BN skeleton with two bulky carbazolyl units | Prevents aggregation, enhanced quenching resistance | 40 | |
BN-TPCzTBA | Twisted heptagonal tribenzo[b,d,f]azepine unit with bulky substituted carbazole unit | High PLQY: 98.6%, asymmetric structure, low efficiency roll-off | 36.8 | |
tPhCzPh3Si | Parent MR-TADF BCz-BN with nonplanar triphenyl silyl and tert-butylphenyl substituents | Suppresses intermolecular aggregation, high PLQY | 34.6 |
MR-TADF Materials
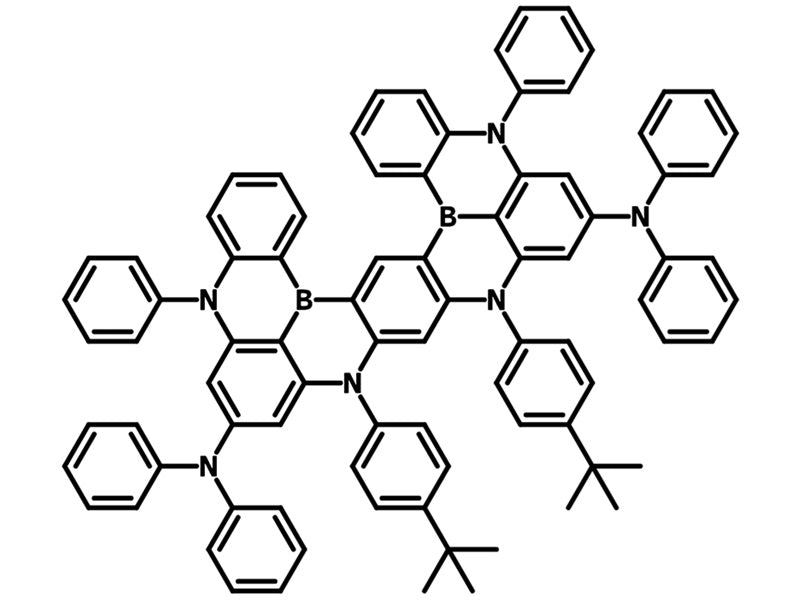
Learn More
References
- Efficient selenium-integrated TADF OLEDs with reduced roll-off, Hu, Y. X. et al., Nature Photonics (2022)
- Peripherally Heavy-Atom-Decorated Strategy Towards High-Performance Pure Green Electroluminescence..., Hu, Y. et al., Angew. Chem. (2023)
- Fused-Nonacyclic Multi-Resonance Delayed Fluorescence Emitter Based on Ladder-Thiaborin..., Nagata, M. et al., Angew. Chem. (2021)
- Understanding and modulating the horizontal orientations and short-range..., Du, M. et al., flexmat (2024)
- Fused π-Extended Multiple-Resonance Induced Thermally Activated Delayed Fluorescence..., Luo, X-F. et al., Advanced Optical Materials (2022)
Further Reading
- Hua et al. (2021); Heavy-atom effect promotes multi-resonance thermally activated delayed fluorescence, Chem. Eng. J., DOI: 10.1016/j.cej.2021.131169.
- Kim et al. (2022); Narrowband Emissive Thermally Activated Delayed Fluorescence Materials, Adv. Opt. Mater., DOI: 10.1002/adom.202201714.
- Luo et al. (2022); Improving reverse intersystem crossing of MR-TADF emitters for OLEDs, J. Semicond., DOI: 10.1088/1674-4926/43/11/110202 .
- Xue et al. (2024); Planar Expansion Enhances Horizontal Orientation of Multi-Resonance TADF Emitter Toward Highly Efficient Narrowband Blue OLEDs, Adv. Funct. Mater., DOI: 10.1002/adfm.202409244.