What is Thermally Activated Delayed Fluorescence (TADF)?
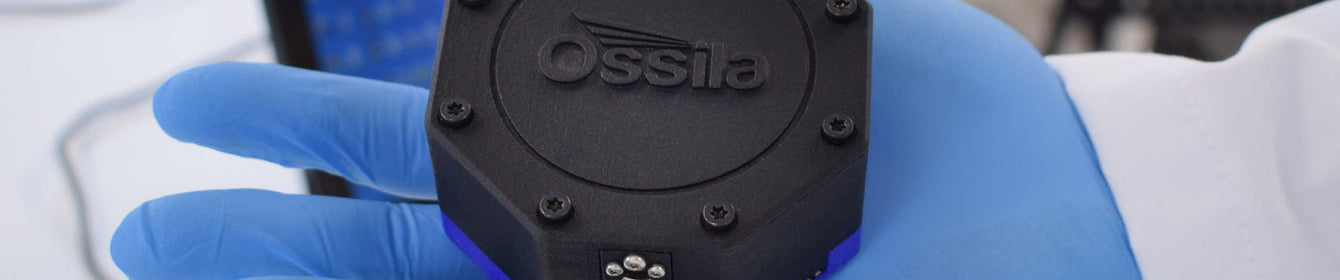
Thermally Activated Delayed Fluorescence (TADF) is a mechanism by which triplet state electrons can be harvested to generate fluorescence. TADF materials (also known as TADF emitters) efficiently convert triplet excitons back to the emissive singlet state through reverse inter-system crossing (RISC). This process requires a small amount of thermal energy (there's usually enough at room temperature). Once the triplet excitons are converted back to the singlet state they recombine and emit light. This process is known as delayed fluorescence.
The maximum internal quantum efficiency (IQE) of organic light-emitting diodes (OLEDs) relies on fluorescence. Efficiency is therefore limited by the amount of triplet states within the system. Because of this, TADF is an important process in materials science to maximise OLED efficiency.
Phosphorescent OLEDs (PHOLEDs) have been used to overcome this problem using organic molecules that have been doped with a heavy metal to facilitate phosphorescence and intersystem crossing. However. it is very difficult to create a stable blue OLED using this approach.
TADF materials promise to unlock new internal quantum efficiencies for OLEDs without the use of heavy metals. Using new blue TADF materials like SPPO13, 2CzPN, and DMAC-DPS, TADF may also allow for the creation of stable blue-emitting-devices.
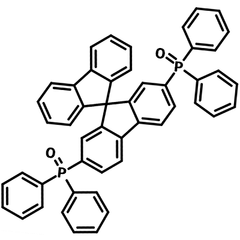
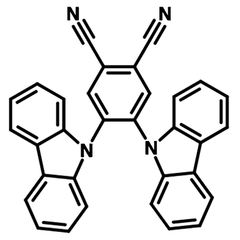
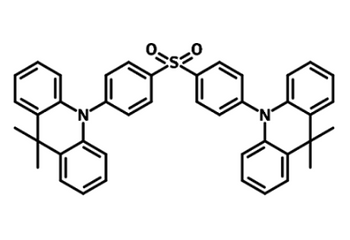
Why TADF OLEDs?
The phrase 'first generation OLED' refers to fluorescent devices, while 'second generation OLED' refers to phosphorescent devices. OLEDs which include TADF materials are therefore known as 'third generation OLEDs'. First generation OLEDs have a maximum internal quantum efficiency of 25%, while second and third generation OLEDs can each have IQEs close to 100%.
Due to the spin selection rules, which dictate that spin must be conserved, when organic molecules are excited optically, these excited states are predominantly singlet states. Essentially, as photons have zero spin, the electron spin must be conserved between the singlet ground state (S0) and the excited state. Hence, occupation of the singlet excited state (S1) dominates.
However, when electrons are excited electrically (as is the case with OLEDs), these spin rules do not apply. Here, a voltage is applied across the organic layer. Electrons are injected into the LUMO (lowest unoccupied molecular orbital) at the cathode and are accelerated towards the anode, where they exit the HOMO (highest occupied molecular orbital). Rather than an ejection of electrons at the anode, this can be thought of as an injection of holes. The holes are accelerated towards the cathode, and when they meet electrons, they couple to form bound electron-hole pairs known as excitons.
Fluorescent OLEDs
Excitons can either be in the singlet or triplet state. This will depend on the relative spins of the electrons as well as which hole they are made up of. In the singlet state, they will recombine to generate fluorescence. However, as there are three triplet states for every singlet state, 75% of the excitons formed are in the triplet state (T1). They are therefore unable to undergo fluorescence, and as phosphorescence is forbidden (though it can still occur at a very low rate). As the triplet excitons are unable to fluoresce, this energy is lost.
The internal quantum efficiency (IQE, ratio of energy output to energy input) of OLEDs that rely on fluorescence is therefore limited to 25%. These are known as first-generation OLEDs.
Phosphorescent OLEDs
To increase the IQE, research that focused on the development of OLEDs that rely on phosphorescence.These second-generation materials incorporate a heavy metal (such as iridium or platinum) into the organic molecule. As a result, we see a large increase in the spin-orbit coupling between the electron spin angular momentum and the orbital angular momentum. This has two effects:
- Phosphorescence is no longer forbidden and, therefore, the molecules can emit from the triplet state.
- The rate of intersystem crossing (ISC) between the first excited singlet state and the first excited triplet state is enhanced.
Through these two mechanisms, the internal quantum efficiency of second-generation OLEDs can be near 100%. One drawback of these second-generation materials is the extreme difficulty in producing stable blue OLEDs. For this reason, commercially available blue LEDs are first-generation and are therefore highly inefficient. A great deal of research has therefore gone into (and is going into) third-generation OLEDs that utilise TADF.
TADF OLEDs
In TADF, excitons in the triplet state gain thermal energy from their surroundings in order to undergo reverse ISC. This sees them transition from the excited triplet state to the excited singlet state. As reverse ISC is a relatively slow process. The fluorescence from excitons that were originally in the triplet state occurs a while after the fluorescence from the original singlet excitons, hence the term, delayed fluorescence.
The triplet state is usually at a slightly lower energy than the singlet state. Therefore, TADF requires that this energy gap be as small as possible, and its probability increases massively as the energy gap approaches 25 meV (the thermal energy at room temperature). Additionally, the rate of reverse ISC must be high compared to non-radiative triplet relaxation. If these conditions are met, the IQE of TADF materials can be close to 100%.
Examples of TADF Materials
A further advantage of TADF OLEDs is that TADF materials are generally available at lower prices than fluorescent and phosphorescent molecules. This makes them an attractive, lower cost alternative to both first- and second-generation OLED emitters in all colours. Here are some examples of TADF materials:
TADF Materials
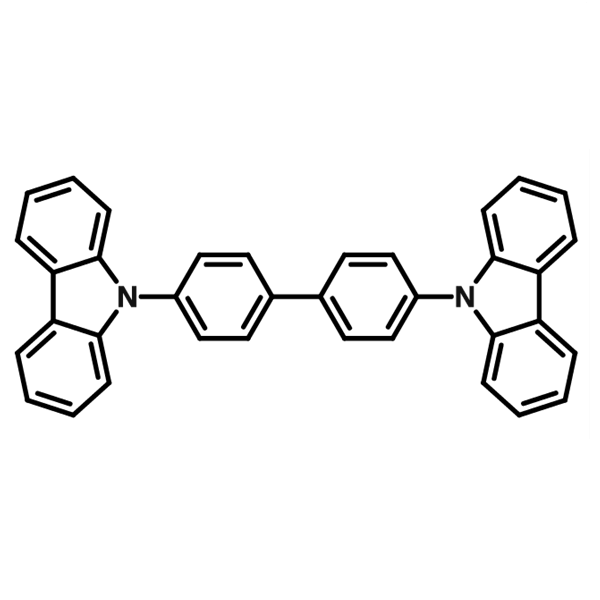
Learn More
Contributing Authors
Written by
PhD Student Collaborator
Edited by
Application Scientist