MR-TADF Applications
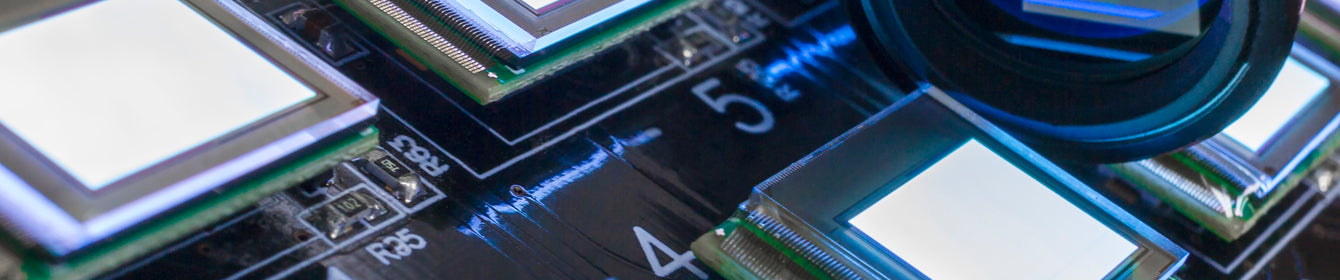
MR-TADF emitters show great application potential in high color purity and high-resolution organic light-emitting diode (OLED) displays, and their long emission lifetimes also make them ideal for use in bioimaging probes, fluorescent sensors, and phototheranostics. The photophysical properties of such emitters, including strong absorption bands in the visible regime and minimal solvatochromism of the emissive excited state, also suggest that these compounds should act as potent photocatalysts.
MR-TADF materials exploit a design strategy where the separation of electron-donating and electron-accepting units within a single molecule minimizes energy losses and enhances exciton utilization. This makes them particularly attractive for use in organic light-emitting diodes (OLEDs) and other advanced technologies.
MR-TADF Display Technologies: Revolutionizing OLEDs
High-Resolution Displays
One of the most promising applications of MR-TADF materials is in OLED displays. Their narrowband emission results in a reduced overlap of emission spectra among red, green, and blue subpixels, leading to exceptional color purity and a wider color gamut. This capability is particularly critical for high-resolution displays used in smartphones, televisions, and virtual reality (VR) devices.
Among MR-TADF emitters, the state-of-the-art ν-DABNA features a fully resonating extended π-conjugated structure. It consists of five benzene rings linked by two boron and four nitrogen atoms, along with two diphenylamino substituents. ν-DABNA exhibits sharp sky-blue electroluminescence at 469 nm (CIE y = 0.11, FWHM = 14 nm) and achieves a maximum EQE of 35% in TADF-OLEDs.
Modifying the nitrogen in the skeleton to oxygen yields the pure blue emitter NO-DBMR, while replacing diphenylamino with carbazole creates the bluish-green emitter Cz-DBMR, both retaining narrow FWHM of 14 nm. Carbazole in Cz-DBMR enhances molecular rigidity and charge localization, boosting the multiple resonance effect. Both emitters show a small ΔEST (0.04 eV), PLQY > 80%, and fast kRISC. OLEDs using NO-DBMR and Cz-DBMR achieve high resolution with excellent color purity and EQEmax of 33.7% and 29.8%, respectively.
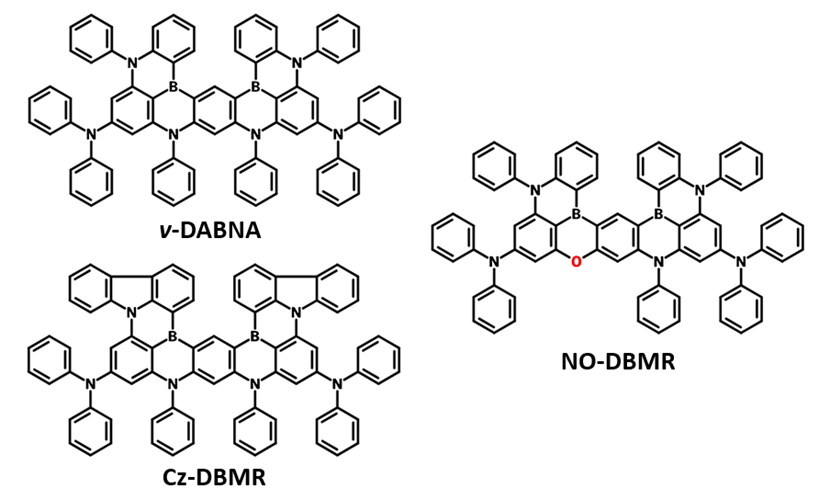
Improving Energy Efficiency
MR-TADF emitters show alternative frontier molecular orbital (FMO) distributions on atoms in the same π-conjugated scaffold with reduced small ΔEST, demonstrating high PLQY and narrow FWHM of 30 nm with sharp emission spectra and low efficiency roll-off. Novel asymmetric MR-TADF emitter 2Cz-PTZ-BN, achieved by molecular design and modification of its parent molecule BCz-BN with extended π-skeleton and peripheral protection, demonstrates a high PLQY of 96% and a fast kRISC of above 1.0×105 s−1. OLED devices based on 2Cz-PTZ-BN show pure green emission with an outstanding EQE of up to 32.8 % without utilizing any sensitizing hosts. Moreover, a high EQE exceeding 23 % was maintained at a high luminance of 1000 cd m−2, representing the highest value for reported green MR-TADF materials at the same luminescence.
Increasing Device Stability
MR-TADF materials exhibit strong molecular rigidity due to their well-defined multi-resonance structures. This enhances thermal and photochemical stability, addressing a common limitation of conventional TADF emitters. Stable emitters are essential for ensuring the longevity of OLED panels, especially in demanding applications like automotive displays.
In OLED devices, low reverse intersystem crossing rate usually restricts the release of the triplet excitons with high energy. The long-lived triplet excitons would easily lead to the triplet–triplet annihilation (TTA) or triplet-polaron annihilation (TPA) between triplet excitons or between triplet excitons and polarons. Under the circumstances, these emitters are prone to chemical decomposition in the high-energy excited states for the formation of TTA or TPA, which heavily impact the stability of the OLEDs.
With the molecular design strategies of extension of core π-skeleton and the incorporation of heave atoms, MR-TADF materials can achieve fast reverse intersystem crossing rates to reduce the triplet’s population and low efficiency roll-offs to reduce the use of higher current densities for high brightness and improvement in device operational lifetime. π-CzBN, a novel double boron-embedded multiple resonance emitter by collocating carbazole building blocks with ν-DABNA heteroatom alignment, achieves an exceptional efficiency of 39.3 % with a narrow full width at half maximum of 21 nm/106 meV in the bluish-green region. π -CzBN shows a fast reverse intersystem crossing rate of 2.9×105 s−1 and the optimized OLED using π-CzBN as the emitter achieves a low efficiency roll-off of 20 % at 1000 cd m−2 and a narrowband emission at 495 nm.
MR-TADF as Photocatalysts
Photocatalysis involves using a photocatalyst to transfer electrons or energy to an organic substrate by absorbing photons, thereby harnessing its excited electronic state. MR-TADF materials are an emerging class of photocatalysts that leverage unique electronic and photophysical properties for diverse applications. By utilizing thermally activated delayed fluorescence (TADF), they efficiently harvest both singlet and triplet excitons, thanks to a small singlet-triplet energy gap (ΔEST) that facilitates reverse intersystem crossing (RISC). This mechanism enhances quantum yield, minimizes energy losses, and boosts photocatalytic efficiency.
While organometallic complexes like Ru(II) and Ir(III) have been widely used as photocatalysts, their reliance on scarce and toxic noble metals makes them expensive. MR-TADF emitters, characterized by short-range charge transfer (SRCT) states, exhibit small ΔEST, narrow emission profiles, small Stokes shifts, high molar absorption efficiencies, and reduced solvatochromism compared to donor-acceptor TADF compounds, making them ideal for photocatalysis.
Electron Transfer Photocatalysis
MR-TADF photocatalysts are extensively utilized in driving photoredox reactions that form the cornerstone of modern organic synthesis. Their high photonic efficiencies and tuneable redox potentials enable the activation of a wide range of substrates. MR-TADF catalysts have shown exceptional performance in promoting carbon-carbon bond forming reactions, such as:
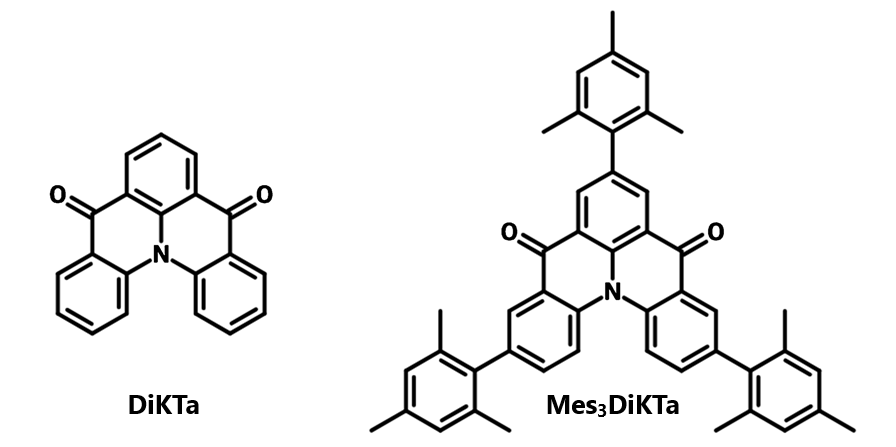
- Cross-couplings
- Cycloadditions
- Radical-mediated transformations
These reactions are critical in constructing complex organic molecules used in pharmaceuticals, agrochemicals, and materials science. The high precision of MR-TADF systems facilitates the selective activation of substrates, enabling transformations like oxidation of alcohols to aldehydes or reductions of nitroarenes to anilines under mild conditions.
MR-TADF materials DiKTa and its mesitylated derivative Mes3DiKTa, with redox potentials similar to 4CzIPN, have been used as photocatalysts for reductive and oxidative quenching reactions. a decarboxylative photo-Giese reaction using N-Cbz protected proline and diethyl maleate under 440 nm LED irradiation, DiKTa and Mes3DiKTa achieved NMR yields of 95% and 91%, comparable to 4CzIPN. In an atom transfer radical addition (ATRA) reaction involving perfluorobutyl iodide and tert-butyl N-allylcarbamate, these photocatalysts yielded 97% and 93%, respectively.
Other MR-TADF emitters such as, DABNA-1, tDABNA, and CzBN have also been applied as photocatalysts in the coupling reaction of benzaldehyde to afford 1,2-diphenyl-1,2-ethanediol under the illumination of 440 nm LEDs in THF at room temperature. A NMR yield of 70% is obtained by using CzBN as the photocatalyst, and lower yields of 64% and 61% for DABNA-1and tDABNA, respectively.
Energy Transfer Photocatalysis
Apart from being photoinduced by the electron transfer process, the photocatalysis can also be induced by Dexter energy transfer (DET), a process known as photoinduced energy transfer (PEnT). One of the most studied examples of PEnT processes is the (E)/(Z) isomerization of alkenes. The crucial deciding factor for efficient Dexter PEnT reactions is the degree of spectral overlap between the emission of the photocatalyst and the spin-forbidden absorption of the substrate. In this mechanism, photocatalyst sensitizes the substrate to an excited state through Förster or Dexter energy transfer. The Förster energy transfer occurs between the excited singlet (S1) states and the Dexter energy transfer predominantly occurs between the excited triplet (T1) states.
A Z/E ratio of 92 : 8 stilbene is achieved by using TADF emitter 4CzIPN as the photocatalyst for the isomerization reaction of (E)-stilbene under the illumination of 440 nm in dichloroethane at room temperature, while Z/E ratios of 59 : 41 and 61 : 39 are observed with DiKTa and Mes3DiKTa, respectively. Also as the photocatalyst, DABNA-1 gives a Z/E ratio of 76 : 24 with cinnamyl acetate as the substrate in THF after 24 h under an inert atmosphere, while tDABNA, CzBN result in Z/E ratios of 70 : 30 and 80/20 under the illumination wavelength of 440 nm.
MR-TADF in Bioimaging: High-Resolution Fluorescent Probes
In the biomedical field, MR-TADF materials are being developed as fluorescent probes for high-resolution bioimaging. Their narrowband emission and high brightness enhance the clarity and precision of imaging, making them ideal for visualizing cellular and molecular structures. As TADF materials generate long-lived emission, time-resolved fluorescence imaging can be used to obtain images with high signal-to-noise ratios against background biological autofluorescence. They provide high photostability and low cytotoxicity, making them suitable for prolonged imaging studies and live cell applications. With their high photoluminescence quantum yield and superior narrowband emission, MR-TADF are great candidates for high-performance biological probes, especially MR-TADF red emitters.
Deep-Tissue Imaging
The long emission wavelengths of certain MR-TADF materials enables them to penetrate deeper into biological tissues, making non-invasive imaging techniques possible. This is particularly advantageous for applications such as tracking cancer progression or studying deep-seated biological processes. The delayed fluorescence of TADF materials improves imaging sensitivity and contrast by minimizing background noise from biological tissues. This enables precise detection of low-abundance biomolecules and subtle biological changes, crucial for early disease detections. By decorating DiKTa core with di([1,1’-biphenyl]-4-yl)amine (PhDPA) and bis(4-methoxyphenyl)amine (MeODPA), orange and red MR-TADF emitters, PhDPA-DiKTa and MeODPA-DiKTa are obtained. Water-dispersible glassy organic dots (g-Odots) based on PhDPA-DiKTa and MeODPA-DiKTa with mCP host and an amphiphilic DSPE-PEG2k polymer show red emissions at 618 nm and 663 nm, with a τd of 203.9 μs for PhDPA-DiKTa g-Odots and 131.6 μs for MeODPA-DiKTa g-Odots, respectively. Consequently, those MR-TADF g-Odots are successfully employed as biocompatible imaging probes of HeLa cells with high color purity and improved imaging performance.
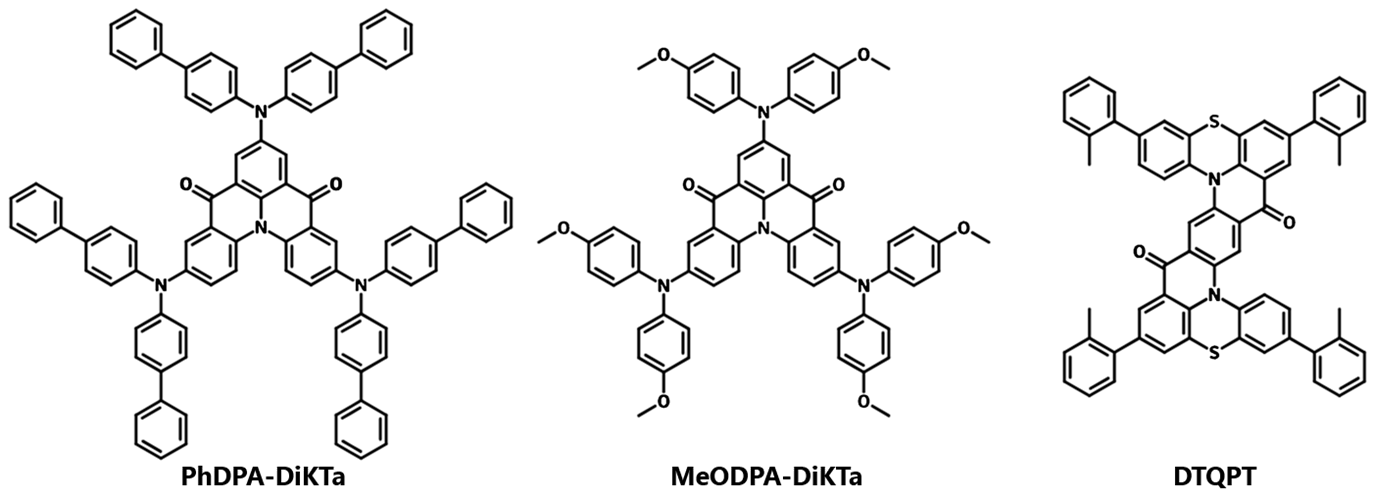
Low Cytotoxicity
Unlike some traditional fluorescent dyes and quantum dots, MR-TADF materials can be designed to exhibit low cytotoxicity, making them safer for in vivo applications. MR-TADF molecules exhibit higher energy conversion efficiency, long-lived triplet excitons, tuneable photophysical properties, and a small singlet-triplet energy gap. This mechanism facilitates efficient intersystem crossing and reverse intersystem crossing with enhanced singlet oxygen generation. As metal-free luminophores, they offer benefits such as reduced health risks, high structural flexibility, and biocompatibility, which can significantly enhance photodynamic therapy treatment efficacy.
Anti-Kasha dual-emission MR-TADF emitter 11-(3,7-di-o-tolyl-10H-phenothiazin-10-yl)-9-oxo-3,7-di-o-tolyl-9H-quinolino[3,2,1-kl]phenothiazine-9,12-dione (DTQPT) can be efficiently endocytosed into the HeLa cells and dual emission imaging in living cells as water-dispersed nanoparticles. MR-TADF emitter DTQPT presents low cytotoxicity with > 85% HeLa cells cell viability retained while being incubated with DTQPT nanoparticles for 24 h.
MR-TADF Photovoltaics: Enhancing Solar Cell Efficiency
While MR-TADF materials are primarily known for their optoelectronic applications in OLEDs, their unique electronic properties make them intriguing candidates for use in organic photovoltaic devices. The ability of MR-TADF materials to harvest triplet excitons and upconvert them to singlets can be utilized to enhance the efficiency of organic solar cells. By incorporating MR-TADF layers, it is possible to increase the utilization of absorbed light, improving the overall energy conversion efficiency.
It is well established that the introduction of the third component, using high boiling solvent additive or solid additives could render the molecular packing and crystallization process in the active layer materials in organic solar cells, leading to more ordered arrangement and proper phase separation of the active layers. Also, polymer donors generally have a shorter exciton diffusion distance than non-fullerene acceptors (NFAs) in the active-layer materials, it is crucial to concurrently increase the exciton lifetime of polymer donors and optimizing the active layer morphology for better device performance. By the introduction of a MR-TADF BNS-H1 additive into the benchmark PM6:Y6 system, it not only promotes the molecular packing of acceptor, but also enhances the emission intensity of PM6 component and extends exciton lifetime and diffusion distance of the active layer, leading to an increased power conversion efficiency of 17.4%. Furthermore, device stability of the BNS-H1 treated PM6:Y6 devices is significantly improved.
MR-TADF Laser Technologies: Narrowband Emitters for Coherent Light Sources
MR-TADF materials' sharp emission peaks and high photoluminescence quantum yields make them suitable candidates for organic lasers. These materials can be used to create coherent light sources with applications in spectroscopy, telecommunications, and advanced imaging techniques. The low optical gain thresholds of MR-TADF materials reduce the energy required to achieve lasing, making them more energy-efficient compared to traditional organic laser systems. Moreover, MR-TADF materials can be chemically tailored to emit specific wavelengths, enabling the development of tuneable laser systems for specialized applications.
Both thermally activated delayed fluorescent and phosphorescent materials can effectively utilize triplet excitons, resulting in nearly 100 % internal quantum efficiency. With reduced singlet-triplet energy gaps, TADF emitters could capture both S1 and T1 excitons through an efficient reverse intersystem crossing (RISC) process. However, the charge transfer characters from S1 to S0 greatly suppresses the oscillator strength of TADF molecules, potentially leading to a small emission cross section and affecting light amplification. MR-TADF materials, with spatially separated frontier molecular orbitals, overcome the disadvantage of small oscillator strength of TADF molecules. The multiple resonance effect can cause a relatively high oscillator strength, leading to a largely stimulated emission cross-section, which is an important factor in achieving optical amplification and low-threshold lasing. CzBNPh, a boron/nitrogen multiple-resonance TADF polycyclic framework fusing carbazole units, shows stimulated emission zone effectively separated from its singlet and triplet absorption thus minimizes the singlet-triplet annihilation under long-pulsed excitation ranging from 20 μs to 2.5 ms. CzBNPh demonstrated a low distributed feedback (DFB) lasing threshold of 0.68 μJ cm−2.
MR-TADF Materials
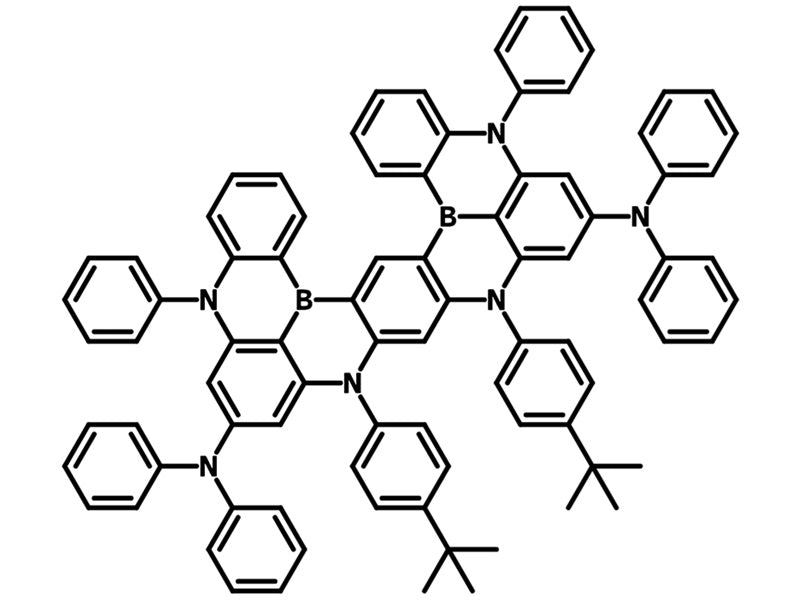
Learn More
References
- Kondo et al. (2019); Narrowband deep-blue organic light-emitting diode featuring an organoboron-based emitter, Nat. Photonics, DOI: 10.1038/s41566-019-0476-5.
- Naveen et al. (2023); Tailoring Extremely Narrow FWHM in Hypsochromic and Bathochromic Shift of Polycyclo-Heteraborin MR-TADF Materials for High-Performance OLEDs, Angew. Chem. Int. Ed., DOI: 10.1002/ange.202306768.
- Jiang et al. (2021); Quenching-Resistant Multiresonance TADF Emitter Realizes 40% External Quantum Efficiency in Narrowband Electroluminescence at High Doping Level, DOI: 10.1002/adma.202106954.