Water-Soluble Fullerenes: Preparation and Applications
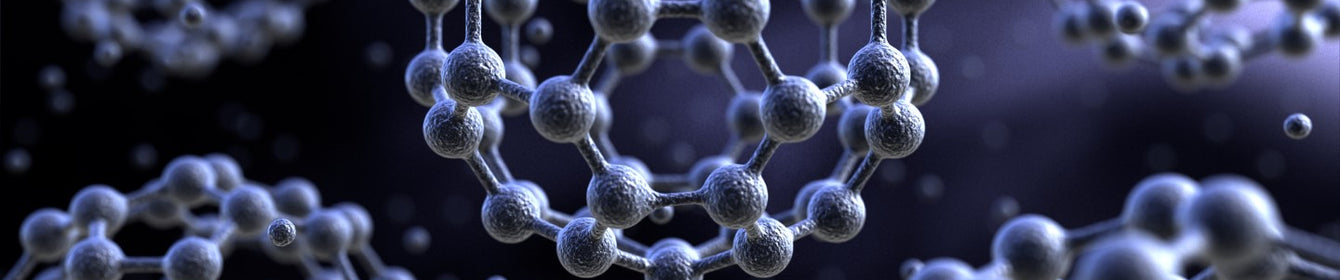
Water-soluble fullerenes, such as fullerenol C60, are either chemically modified, polymer grafted, or encapsulated in a hydrophillic agent. Fullerenes can then disperse or solubilize in aqueous media. Typical examples of water-soluble fullerenes are polyhydroxylated fullerenes, also knowns as fullerenols, polycarboxylated fullerenes and polysulfonated fullerenes.
Fullerenes have attracted interest due to their distinctive properties, including high electron affinity, thermal stability, and chemical reactivity. However, a notable limitation of fullerenes is their hydrophobic nature, which restricts their application in aqueous environments. To overcome this challenge, significant research has been devoted to developing water-soluble fullerenes. These modified fullerenes open new avenues in fields such as electronics, medicine, materials science, and environmental applications.
Preparation of Water-Soluble Fullerenes
The synthesis of water-soluble fullerenes typically involves the functionalization of the fullerene cage with hydrophilic groups. Various approaches have been developed to achieve this and typically involve covalent or non-covalent modifications.
Covalent Functionalization of Fullerenes
Covalent functionalization involves the attachment of hydrophilic groups to the fullerene core through strong covalent bonds. Common strategies include the addition of functional groups such as:
- Hydroxyl (-OH)
- Carboxyl (-COOH)
- Sulfonic acid (-SO3H)
These groups can be introduced through various chemical reactions, such as cycloaddition, Bingel reaction, and radical addition.
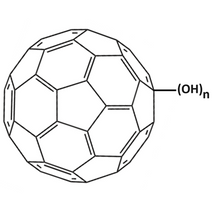
Hydroxylation
The introduction of hydroxyl (-OH) groups to fullerenes can be achieved via reaction with hydrogen peroxide (H2O2) in the presence of catalysts like osmium tetroxide. The resulting polyhydroxylated fullerenes are often termed fullerenols or fullerols. The most famous example being fullerenol C60 which is hydroxylated buckminsterfullerene.
Fullerenols are formed by attaching polar hydroxyl groups to the carbon atoms of the fullerene molecule. This renders it water-soluble and enhances its chemical reactivity. The number and distribution of hydroxyl groups can vary, resulting in different fullerenol derivatives. Such derivatives are commonly denoted as C60(OH)n, where "n" indicates the number of hydroxyl groups attached. The multiple hydroxyl groups are capable of hydrogen bonding with water molecules, hence the water solubility. This contrasts sharply with the hydrophobic nature of pristine fullerenes and is one of the most significant advantages of fullerenol in practical applications.
Carboxylation
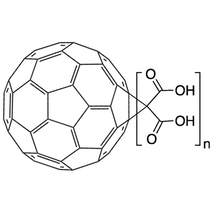
Carboxylated fullerenes can be synthesized through reactions such as Prato reaction, where amino acids or other carboxyl-containing reagents are reacted with fullerenes. The resulting fullerene derivatives exhibit improved solubility in water and can form stable colloidal suspensions.
Fullerene C60 malonic acid is one example of a carboxylated fullerene. It is formed through the covalent attachment of malonic acid groups (-CH2(COOH)2) to a C60 fullerene core. This is typically done via a cyclopropanation reaction known as the Bingel-Hirsch reaction. The number of malonic acid groups attached can vary, leading to different derivatives, such as monoadducts, bisadducts, and higher adducts, each with distinct properties.
As well as enhancing the solubility of the molecule, carboxylic acid groups provide sites for further chemical reactions including, esterification, amidation, and coordination with metal ions. This reactivity makes C60 malonic acid a valuable precursor for further functionalization and the synthesis of complex fullerene-based materials.
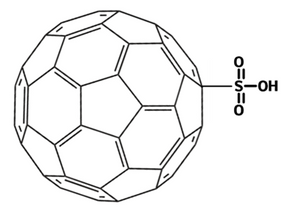
Sulfonation
The sulfonation of fullerenes involves the introduction of sulfonic acid groups through reactions with sulfuric acid or chlorosulfonic acid. Sulfonated fullerenes are highly water-soluble and have been used in various biomedical applications.
Sulfonated fullerene (C60SO3H), with superparamagnetic properties, is potentially applicable for magnetically guided drug delivery. Sulfonated fullerenes with tentacle-like structures demonstrated facile adsorption of water molecules, showing great potential for humidity sensors such as non-contact detection of respiration and soil humidity.
Non-Covalent Functionalization of Fullerenes
Non-covalent functionalization of fullerenes involves the interaction of fullerenes with other molecules through weaker forces such as:
- Van der Waals forces
- π-π stacking
- Hydrogen bonding
This approach often involves the use of surfactants, polymers, or cyclodextrins to encapsulate the fullerene molecules. Non-covalent functionalization allows the fullerene molecules to assemble into well-ordered crystalline nano- or micro-clusters. The unique spherical structures, extended π-system, and excellent solubility of the clusters render fullerenes an excellent 0D or 1D building block.
Cyclodextrin Complexation
Cyclodextrins are cyclic oligosaccharides (polymer of simple sugars) with a hydrophobic cavity that can encapsulate hydrophobic molecules like fullerenes. The exterior of the cyclodextrin is hydrophilic, allowing the complex as a whole to be soluble in water. This method is particularly advantageous as it preserves the electronic properties of the fullerene while rendering it water-soluble.
Host molecules such as γ-cyclodextrin and sulfocalix[8]arene are highly effective at complexing with fullerenes and their derivatives. Mechanochemical high-speed vibration milling (HSVM) is used to facilitate complexation. C60 derivative/γ-cyclodextrin (γ-CDx) complexes demonstrate significantly higher photodynamic activities than those of the pristine C60 under photoirradiation at long wavelengths (610–720 nm). These are the optimal wavelengths for photodynamic therapy (PDT). The γ-CDx-complexed C60 derivatives are soluble in water at high concentrations (i.e., >1.0 mM).
Surfactant Encapsulation
Surfactants can be used to encapsulate fullerene molecules in micelles. The hydrophobic core contains the fullerene and the hydrophilic exterior interacts with water. This method is commonly used to disperse fullerenes in aqueous environments for environmental and biological applications.
Ordered structures of C60 and surfactant Triton X-100 (ratio of 1.7 × 10–3 : 1) form assemblies with nanoscale dimensions (~220 × 75 nm) in water. The rod-like nanostructure is composed of C60 fullerene as the inner and Triton X-100 wrapping around the C60 rod. Triton X-100 encapsulated C60 nanorods have potential applications in cosmetics and drug delivery. The nanostructures have enhanced solubility in aqueous solutions and novel optoelectronic properties, as well as the antioxidant and anti-carcinogenic activity of C60.
Polymer Wrapping
Polymers with hydrophilic backbones can non-covalently wrap around fullerene molecules, providing a water-soluble shell. Polymers like polyethylene glycol (PEG) have been extensively studied for this purpose, as they not only improve solubility but also enhance biocompatibility.
Poly(N-vinylpyrrolidone) (PVP) has known to form water-soluble complexes with fullerenes at high concentrations simply by grinding them together in a mortar. Water-soluble fullerene/PMPCn complexes based on hydrophobic fullerene and hydrophilic and biocompatible poly(2-(methacryloyloxy)ethyl phosphorylcholine) can be obtained by physically mixing fullerenes (C60 or C70) and polymer PMPCn together. Singlet oxygen can be generated upon visible light irradiation (>420 nm) of those water-soluble complexes as photodynamic therapy (PDT) agents.
Water-soluble Fullerenes in Biomedical Applications
The most significant impact of water-soluble fullerenes has been in the field of medicine. Owing to their photochemistry, radical quenching, and hydrophobicity to form unique structures from one to three-dimensional supramolecular complexes, water-soluble organo-fullerenes offer potential for use in drug delivery, photodynamic therapy, and as antioxidants.
Drug Delivery
Water-soluble fullerenes can act as carriers for hydrophobic drugs, improving their solubility and bioavailability. For instance, fullerene-based drug delivery systems have been investigated for the delivery of anticancer drugs, where the fullerene enhances stability and targeted delivery. Biocompatible and water-soluble fluorescent fullerene (C60-TEG-COOH) coated nanoparticles have been used for pH-sensitive drug release and fluorescent cell imaging. The release of doxorubicin hydrochloride (drug) could be triggered under a mild acidic environment (pH = 5.0).
Water-soluble fullerenes can complex with DNA in buffer solution. When these fullerene/siRNA complexes encounter plasma proteins in the bloodstream, they grow into larger particles. These larger particles quickly clog the lung capillaries, releasing siRNA into lung cells to interfere with targeted cancer genes. They are then rapidly cleared from the lungs.
Photodynamic Therapy (PDT)
Fullerenes generate reactive oxygen species (ROS) upon illumination due to their ability to absorb visible light and efficiently undergo intersystem crossing to a long-lived triplet state. This means fullerenes act as photosensitizers, making them suitable for use in photodynamic therapy (PDT). PDT is a technique used to treat cancer by producing ROS near tumours in order to kill them. Iron oxide nanoparticles decorated fullerene (C60-IONP-PEG) demonstrates a strong superparamagnetism and powerful PDT capacity.
As a photosensitizer, fullerene can be easily modified with light harvesting functional groups. The modified fullerene molecules can self-assemble into supramolecular aggregates. They can be components within larger nanoparticles that are both therapeutic and diagnostic. For example, C60-copolymer (polyethylene glycol methyl ether methacrylate and 2-aminoethylmethacrylamide) conjugates show a strong photodynamic therapy effect on human lung cancer cells (A549) under light irradiation (450 nm).
Antioxidants
Fullerenes have been found to exhibit strong antioxidant properties, capable of scavenging free radicals and protecting cells from oxidative damage. Water-soluble fullerenes, such as fullerenol, have been studied for their potential in treating conditions associated with oxidative stress, including neurodegenerative diseases. The fullerene skeleton shows affinity for hydrophobic enzymatic fragments and lipid structures of cell membranes.
The unsaturated fullerene double bonds, along with the large number of attached −OH groups, also make fullerenol a better radical scavenger. Fullerenols show excellent efficiency in eliminating radicals such as:
- superoxide anion (O2•−)
- hydroxyl (HO•)
- nitric oxide (NO•)
Apart from fullerenols, hexasulfobutyl fullerenes C60(CH2)4SO3H)6 (FC4S) were also among the earliest developed water-soluble fullerene derivatives investigated for radical scavenging.
Environmental Applications of Water-Soluble Fullerenes
Water-soluble fullerenes have also found applications in environmental science, particularly in water treatment and pollution management. Due to their unique properties with high affinity for electrons, fullerenes can be either used together with other semiconducting materials such as titanium dioxide. Fullerene-based materials are great candidates for photocatalytic applications, such as water splitting, heavy metal ions reduction and removal, pollutant degradation and bacterial disinfection.
Water Treatment
Fullerenes show great potentials for water treatment to obtain clean water by the removal of heavy metals, salts, pathogens, chemicals, microorganisms, oils, etc. Water-soluble fullerenol-incorporated thin-film nanocomposite (FTFC) membranes demonstrated 83.03% higher water flux and reverse salt flux selectivity compared with those of pure thin-film composite membrane (TFC).
Pollution Management
Fullerenes can interact with a wide range of pollutants, combined with their water solubility, allows for more efficient clean up processes. For example, Fullerol enhanced the degradation of the antibiotic chloramphenicol (CAP) by about 22 times compared to the standard Fe(III)/H2O2 system. Tetrakis C60 aminofullerenes immobilized on silica support have inactivated virus and bacteria under visible light irradiation by efficiently producing singlet oxygen (1O2).
As a photocatalyst, aminoC60/silica nanocomposite can also effectively degrade pharmaceuticals such as acetaminophen, carbamazepine, cimetidine, propranolol, ranitidine, sulfisoxazole, and trimethoprim as pollutants in the wastewater stream under visible light irradiation faster than common semiconductor photocatalysts.
Uses of Water-Soluble Fullerenes in Material Science
In materials science, water-soluble fullerenes are being explored for their potential in creating new materials with unique properties.
Nanocomposites
Fullerenes can be incorporated into polymers to create nanocomposites with enhanced mechanical, thermal, and electrical properties. The dispersion and solubility of the fullerene molecules in polymers remain as the major challenges during the nanocomposite formation The water solubility of these fullerenes facilitates their incorporation into aqueous polymer solutions, enabling the development of advanced materials for various industrial applications.
Sensors
The electronic properties of fullerenes make them suitable for use in sensors with enhanced sensitivity, selectivity, real-time responsiveness and rapid signal transmission towards various biomolecules. Water-soluble fullerenes can be employed in the development of biosensors for detecting biological molecules, pollutants, or other analytes in aqueous environments. Their sensitivity and selectivity make them valuable tools in environmental monitoring and medical diagnostics.
For example, an electrochemical immunosensor based on fullerene-C60 and copper nanoparticles composite film on glassy carbon electrode exhibited excellent sensitivity, special selectivity, and long-term stability for the detection of prostate-specific antigen (PSA).
Solar Cells
In organic photovoltaics, fullerenes are widely used as electron acceptors. Water-soluble fullerene derivatives have been explored for next-generation solar cells that are compatible with aqueous processing techniques, improving the scalability and environmental friendliness of solar cell manufacturing.
A monolayer of fullerenol as the interface between perovskite and TiO2 electron transport layer can dramatically facilitate the charge extraction and transportation and decreases the interfacial resistance. The resulting n-i-p perovskite solar cell device demonstrated a higher power conversion efficiency (PCE) of 14.69%, which is ∼17.5% improvement compared with that (12.50%) of the control device without the fullerenol interlayer.
The incorporation of C60-2NH3 as an interlayer between a FA0.6MA0.4Pb0.7Sn0.3I3 perovskite and a C60 fullerene layer enabled superior band energy alignment between the perovskite and C60 acceptor, reduced non-radiative recombination, and enhanced carrier mobility.
Limitations of Water-Soluble Fullerenes
Despite the promising applications of water-soluble fullerenes, several challenges remain. The toxicity of fullerenes, particularly in biomedical applications, is a concern that requires further investigation. While functionalization can improve biocompatibility, comprehensive studies on the long-term effects of fullerene exposure are needed.
The scalability of synthesis methods for water-soluble fullerenes poses another challenge. Current methods are often complex and costly, limiting the commercial viability of these materials. Developing more efficient and cost-effective synthesis techniques will be crucial for the broader adoption of water-soluble fullerenes in various industries.
Future research is likely to focus on optimizing the functionalization methods to improve the solubility, stability, and biocompatibility of fullerenes. Additionally, the exploration of new applications, particularly in emerging fields such as nanomedicine and environmental remediation, will continue to drive interest in these fascinating materials.
Fullerenol C60
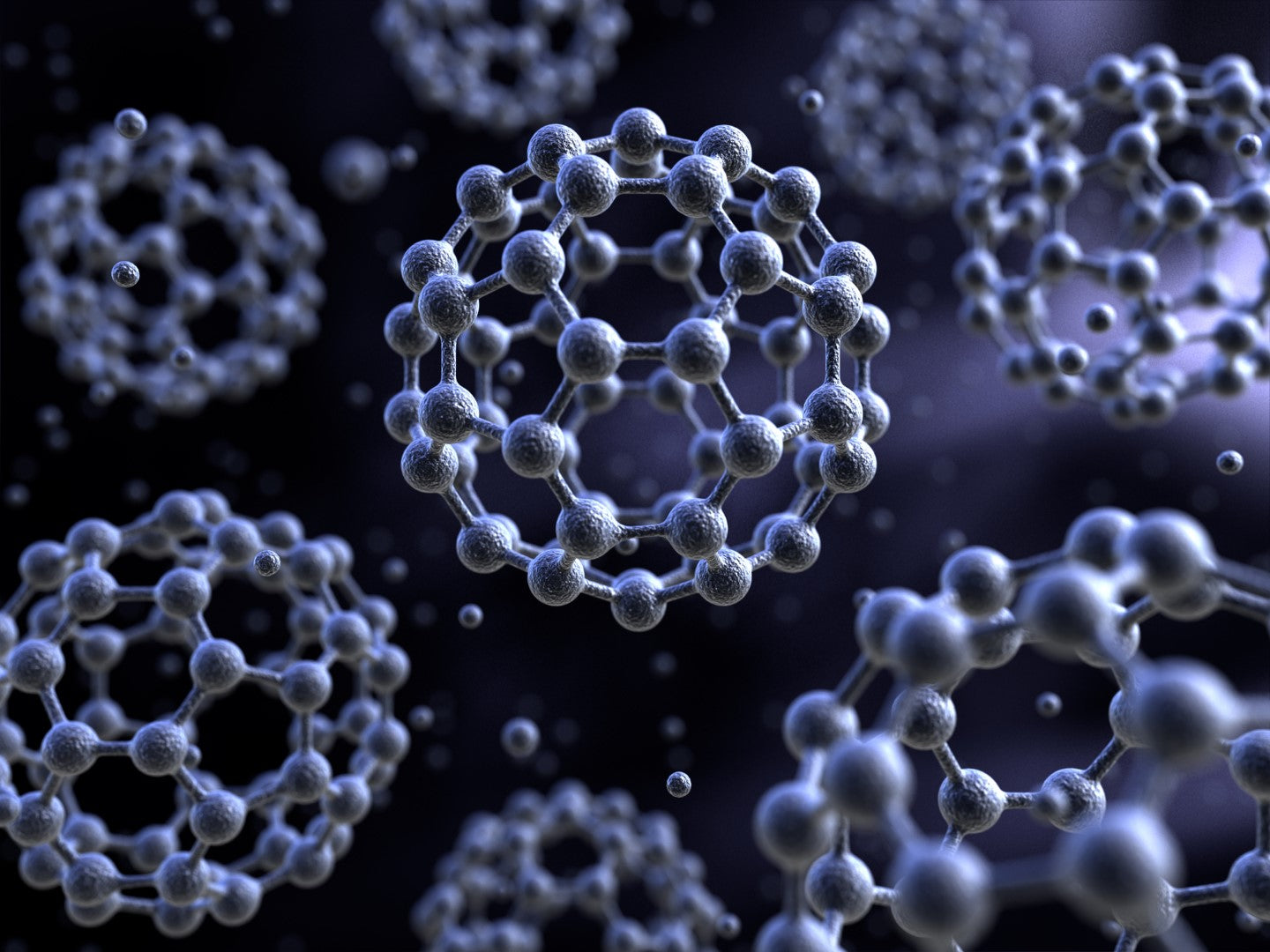
Learn More
Further Reading
- Grebowski et al. Antioxidant activity of highly hydroxylated fullerene C60 and its interactions with the analogue of α-tocopherol, Free Radic. Biol. Med.(2020)
- Nakagawa et al. Cytotoxic effects of hydroxylated fullerenes on isolated rat hepatocytes via mitochondrial dysfunction, Arch. Toxicol. (2011)
- Kokubo et al. Facile and scalable synthesis of a highly hydroxylated water-soluble fullerenol as a single nanoparticle. Nano Res. 4, (2010)